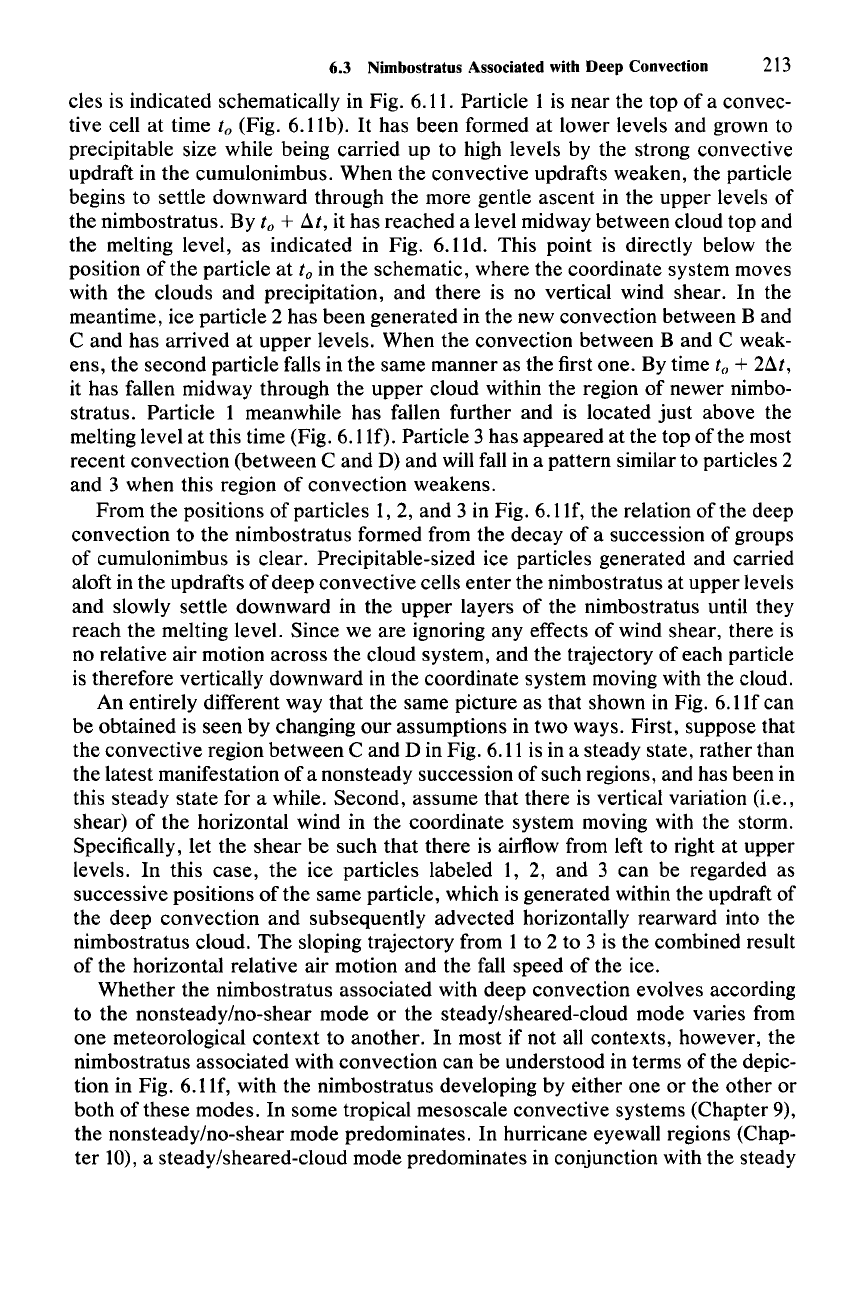
6.3 Nimbostratus Associated with Deep Convection
213
cles is indicated schematically in Fig. 6.11. Particle 1 is near the top of a convec-
tive cell at time
to (Fig. 6.11b).
It
has been formed at lower levels and grown to
precipitable size while being carried up to high levels by the strong convective
updraft in the cumulonimbus. When the convective updrafts weaken, the particle
begins to settle downward through the more gentle ascent in the upper levels of
the nimbostratus. By
to +
at,
it has reached a level midway between cloud top and
the melting level, as indicated in Fig. 6.11d. This point is directly below the
position of the particle at
to in the schematic, where the coordinate system moves
with the clouds and precipitation, and there is no vertical wind shear. In the
meantime, ice particle 2 has been generated in the new convection between
Band
C and has arrived at upper levels. When the convection between
Band
C weak-
ens, the second particle falls in the same manner as the first one. By time
to
+
2at,
it has fallen midway through the upper cloud within the region of newer nimbo-
stratus. Particle 1 meanwhile has fallen further and is located just above the
melting level at this time (Fig. 6.11f). Particle 3 has appeared at the top of the most
recent convection (between C and D) and will fall in a pattern similar to particles 2
and 3 when this region of convection weakens.
From the positions of particles 1, 2, and 3 in Fig. 6.11f, the relation of the deep
convection to the nimbostratus formed from the decay of a succession of groups
of cumulonimbus is clear. Precipitable-sized ice particles generated and carried
aloft in the updrafts of deep convective cells enter the nimbostratus at upper levels
and slowly settle downward in the upper layers of the nimbostratus until they
reach the melting level. Since we are ignoring any effects of wind shear, there is
no relative air motion across the cloud system, and the trajectory of each particle
is therefore vertically downward in the coordinate system moving with the cloud.
An entirely different way that the same picture as that shown in Fig. 6.11f can
be obtained is seen by changing our assumptions in two ways. First, suppose that
the convective region between C and D in Fig. 6.11 is in a steady state, rather than
the latest manifestation of a nonsteady succession of such regions, and has been in
this steady state for a while. Second, assume that there is vertical variation (i.e.,
shear) of the horizontal wind in the coordinate system moving with the storm.
Specifically, let the shear be such that there is airflow from left to right at upper
levels. In this case, the ice particles labeled 1, 2, and 3 can be regarded as
successive positions of the same particle, which is generated within the updraft of
the deep convection and subsequently advected horizontally rearward into the
nimbostratus cloud. The sloping trajectory from 1 to 2 to 3 is the combined result
of the horizontal relative air motion and the fall speed of the ice.
Whether the nimbostratus associated with deep convection evolves according
to the nonsteady/no-shear mode or the steady/sheared-cloud mode varies from
one meteorological context to another. In most if not all contexts, however, the
nimbostratus associated with convection can be understood in terms of the depic-
tion in Fig. 6.1lf, with the nimbostratus developing by either one or the other or
both of these modes. In some tropical mesoscale convective systems (Chapter 9),
the nonsteady/no-shear mode predominates. In hurricane eyewall regions (Chap-
ter 10), a steady/sheared-cloud mode predominates in conjunction with the steady