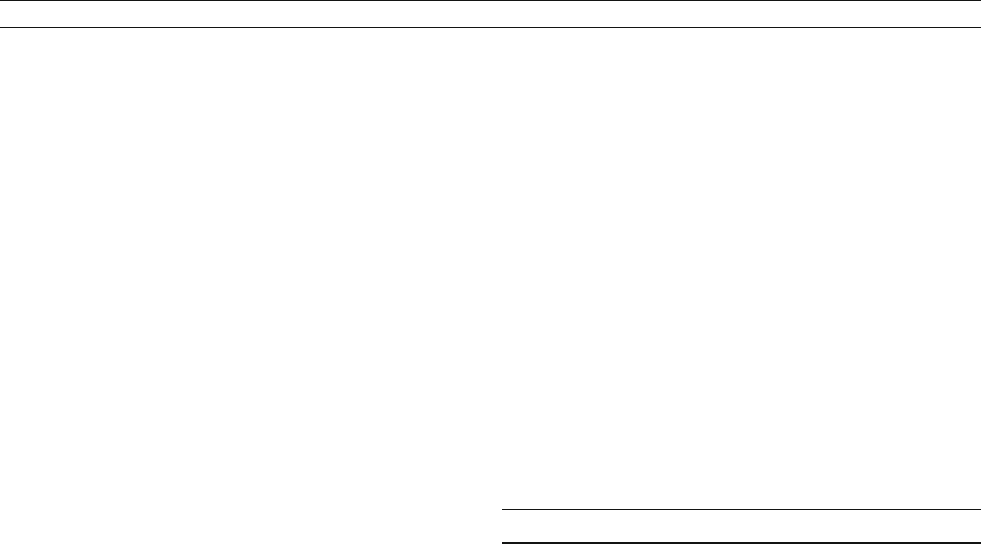
Blodgett, T.A., Lenters, J.D., and Isacks, B.L., 1997. Constraints on
the origin of paleolake expansions in the central Andes. Earth
Interactions, 1,1–28.
Bradley, R.S., 1999. Paleoclimatology. San Diego, CA: Academic Press,
613pp.
Cross, S.L., Baker, P.A., Seltzer, G.O., Fritz, S.C., Dunbar, R.B., 2001.
Late Quaternary climate and hydrology of tropical South America
inferred from an isotopic and chemical model of Lake Titicaca, Bolivia
and Peru. Quaternary Res., 56,1–9.
DeMenocal, P., Ortiz, J., Guilderson, T., Sarnthein, M., 2000. Coherent
high- and low-latitude climate variability during the Holocene warm
period. Science, 288, 2198–2202.
Fleitmann, D., Burns, S., Mudelsee, M., Neff, U., Kramers, J., Mangini, A.,
and Matter, A., 2003. Holocene forcing of the Indian monsoon recorded
in a stalagmite from Southern Oman. Science, 300, 1737–1739.
Gasse, F., Barker, P., Gell, P.A., Fritz, S.C., Chalie, F., 1997. Diatom-
inferred salinity in palaeolakes: An indirect tracer of climate change.
Quaternary Sci. Rev., 16, 547–563.
Haberle, S., and Maslin, M., 1999. Late Quaternary vegetation and climate
changes in the Amazon basin based on a 50,000 year pollen record from
the Amazon Fan ODP Site 932. Quaternary Res., 51,27–38.
Harris, S.E., Mix, A.C., 1999. Pleistocene precipitation balance in
the Amazon Basin recorded in deep sea sediments. Quaternary Res.,
51,14–26.
Haug, G., Hughen, K., Sigman, D., Peterson, L., Rohl, U., 2001. South-
ward migration of the Intertropical Convergence Zone through the
Holocene. Science, 293, 1304–1308.
Hoffmann, G., et al., 2003. Coherent isotope history of Andean ice cores
over the last century. Geophys. Res. Lett., 30, 10.1029/ 2002GL014870.
Hu, F.S., and Shemesh, A., 2003. A biogenic-silica delta O-18 record of
climatic change during the last glacial-interglacial transition in
southwestern Alaska. Quaternary Res., 59, 379–385.
Huang, Y., Shuman, B., Wang, Y., and Webb, III, T., 2004. Hydrogen
isotope ratios of individual lipids in lake sediments as novel tracers
of climatic and environmental change: A surface sediment test.
J. Paleolimnol., 31, 363–375.
Kastner, T.P., and Goñi, M.A., 2003. Constancy in the vegetation of
the Amazon Basin during the Late Pleistocene: Evidence from
the organic matter composition of Amazon Deep Sea Fan sediments.
Geology, 31
, 291–294.
Kull, C., and Grosjean, M., 2000. Late Pleistocene climate conditions in the
north Chilean Andes drawn from a climate-glacier model. J. Glaciol.,
46, 622–632.
Maher, B.A., and Thompson, R., 1995. Paleorainfall reconstructions
from pedogenic magnetic susceptibility variations in Chinese loess.
Quaternary Res., 44, 383–391.
Maley, J., 1996. The African rainforest – main characteristics of changes in
vegetation and climate from the Upper Cretaceous to the Quaternary.
Proc. R. Soc. Edinb., 104B,31–73.
Mann, M.E., 2002. The value of multiple proxies. Science, 297,
1481–1482.
Maslin, M.A., and Burns, S.J., 2000. Reconstruction of the Amazon
basin effective moisture availability over the past 14,000 years. Science,
290, 2285–2287.
McCulloch, M.T., Gagan, M.K., Mortimer, G.E., Chivas, A.R., and Isdale,
P.J., 1994. A high resolution Sr /Ca and d
18
O coral record from the
Great Barrier Reef, Australia, and the 1982–83 El Niño. Geochim. Cos-
mochim. Acta, 58, 2747–2754.
Peterson, L., Haug, G., Hughen, K., and Rohl, U., 2000. Rapid changes in
the hydrologic cycle of the tropical Atlantic during the last glacial.
Science, 290, 1947–1951.
Rozanski, K., Araguas-Araguas, L., and Gonfiantini, R., 1993. Isotopic
patterns in modern global precipitation. In Swart, P.K., Lohmann,
K.C., MacKenzie, J., and Savin, S. (eds.), Climate Change in Continen-
tal Isotopic Records. Washington D.C: American Geophysical Union.
AGU Monograph series, no. 78, pp. 1–37.
Schwalb, A., 2003. Lacustrine ostracodes as stable isotope recorders
of late-glacial and Holocene environmental dynamics and climate.
J. Paleolimnol., 29, 265–351.
Schwarcz, H., and Yonge, C., 1983. Isotopic composition of palaeowaters
as inferred from speleothem and its fluid inclusions. In anon. (ed.),
Palaeoclimates and Palaeowaters; a Collection of Environmental
Isotope Studies. Vienna, Austria: International Atomic Energy Agency,
pp. 115–133.
Seltzer, G., Rodbell, D., and Burns, S., 2000. Isotopic evidence for
late Quaternary climatic change in tropical South America. Geology,
28,35–38.
Villalba, R., Grau, H.R., Boninsegna, J.A., Jacoby, G.C., and Ripalta, A.,
1998. Tree-ring evidence for long-term precipitation changes in sub-
tropical South America. Int. J. Climatol., 18, 1463–1478.
Cross-references
Dendroclimatology
Diatoms
Ice Cores, Mountain Glaciers
Lacustrine Sediments
Lake Level Fluctuations
Loess Deposits
Ostracodes
Paleoclimate Proxies, an Introduction
Paleosols, Pre-Quaternary
Pollen Analysis
Stable Isotope Analysis
Speleothems
Uranium-Series Dating
PALEOSOLS, PRE-QUATERNARY
Introduction and definitions
Paleopedology is the study of the genesis, properties, climate, and
landscape records of fossil soils, or paleosols. Pre-Quaternary
paleosols are buried, “fossilized” soil horizons, which are older
than 2 Ma; they are also commonly lithified, i.e., converted from
soil into rock by the geological processes of burial diagenesis
(Retallack, 1991). Unlike the more rigid definition used by soil
scientists, who consider a soil to be a body of geologic material
that supports plant life, geoscientists interpret pre-Quaternary
paleosols as any former subaerially exposed surfaces or layers
of earth material that have been affected by physical, chemical,
and biological weathering processes. However, evidence for
the presence of plants is not considered a prerequisite for
identification of a fossil soil (Retallack, 2001). This broader
geological definition allows for greater flexibility in examining
parts of Earth history during which terrestrial plants were
not present.
Paleosol features
Impetus for the study of pre-Quaternary paleosols largely origi-
nated with Dr. Gregory Retallack at the University of Oregon,
who has published a widely used textbook on paleopedology
(Retallack, 2001). In a 1985 Geological Society of America Pen-
rose Conference on paleosols (organized by Dr. Retallack), geos-
cientists, for the first time, began to think about soils of the
past set in the backdrop of “deep” geologic time. Recognition
and interpretation of pre-Quaternary paleosols requires the use
of multidisciplinary approaches that involve integration of field
morphological, microscopic, and geochemical data, as well as an
appreciation for evolutionary changes in terrestrial biota and flora
that have occurred over time (Mora and Driese, 1999). Field mor-
phological features such as plant root traces (in post-Silurian
paleosols), soil horizons, and soil structures (especially peds or
natural soil aggregates), are very diagnostic for pre-Quaternary
paleosol identification (Figure P30a;Retallack,1988). Micromor-
phological (thin section) features are commonly well preserved in
748 PALEOSOLS, PRE-QUATERNARY