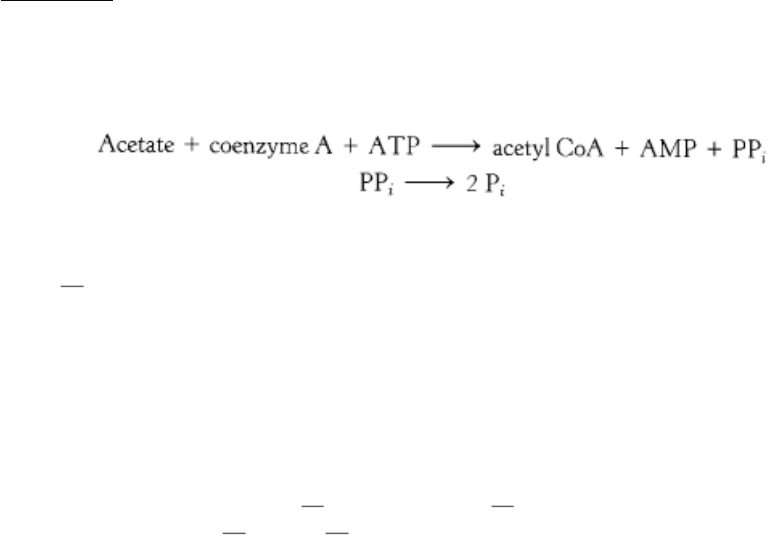
while oxidizing biosynthetic reducing power, NADPH, to NADP
+
. Because it uses oxygen, this pathway generates free
radicals that damage tissues. Moreover, because the system consumes NADPH, the antioxidant glutathione cannot be
regenerated (Section 20.5), exacerbating the oxidative stress.
What are the effects of the other metabolites of ethanol? Liver mitochondria can convert acetate into acetyl CoA in a
reaction requiring ATP. The enzyme is the thiokinase that normally activates short-chain fatty acids.
However, further processing of the acetyl CoA by the citric acid cycle is blocked, because NADH inhibits two important
regulatory enzymes
isocitrate dehydrogenase and α-ketoglutarate dehydrogenase. The accumulation of acetyl CoA
has several consequences. First, ketone bodies will form and be released into the blood, exacerbating the acidic condition
already resulting from the high lactate concentration. The processing of the acetate in the liver becomes inefficient,
leading to a buildup of acetaldehyde. This very reactive compound forms covalent bonds with many important functional
groups in proteins, impairing protein function. If ethanol is consistently consumed at high levels, the acetaldehyde can
significantly damage the liver, eventually leading to cell death.
Liver damage from excessive ethanol consumption occurs in three stages. The first stage is the aforementioned
development of fatty liver. In the second stage
alcoholic hepatitis groups of cells die and inflammation results. This
stage can itself be fatal. In stage three cirrhosis fibrous structure and scar tissue are produced around the dead cells.
Cirrhosis impairs many of the liver's biochemical functions. The cirrhotic liver is unable to convert ammonia into urea,
and blood levels of ammonia rise. Ammonia is toxic to the nervous system and can cause coma and death. Cirrhosis of
the liver arises in about 25% of alcoholics, and about 75% of all cases of liver cirrhosis are the result of alcoholism. Viral
hepatitis is a nonalcoholic cause of liver cirrhosis.
III. Synthesizing the Molecules of Life 30. The Integration of Metabolism
Summary
Metabolism Consists of Highly Interconnected Pathways
The basic strategy of metabolism is simple: form ATP, reducing power, and building blocks for biosyntheses. This
complex network of reactions is controlled by allosteric interactions and reversible covalent modifications of enzymes
and changes in their amounts, by compartmentation, and by interactions between metabolically distinct organs. The
enzyme catalyzing the committed step in a pathway is usually the most important control site. Opposing pathways such
as gluconeogenesis and glycolysis are reciprocally regulated so that one pathway is usually quiescent when the other is
highly active.
Each Organ Has a Unique Metabolic Profile
The metabolic patterns of the brain, muscle, adipose tissue, kidney, and liver are very different. Glucose is essentially the
sole fuel for the brain in a well-fed person. During starvation, ketone bodies (acetoacetate and 3-hydroxybutyrate)
become the predominant fuel of the brain. Adipose tissue is specialized for the synthesis, storage, and mobilization of
triacylglycerols. The kidney produces urine and reabsorbs glucose. The diverse metabolic activities of the liver support
the other organs. The liver can rapidly mobilize glycogen and carry out gluconeogenesis to meet the glucose needs of
other organs. It plays a central role in the regulation of lipid metabolism. When fuels are abundant, fatty acids are
synthesized, esterified, and sent from the liver to adipose tissue. In the fasting state, however, fatty acids are converted
into ketone bodies by the liver.