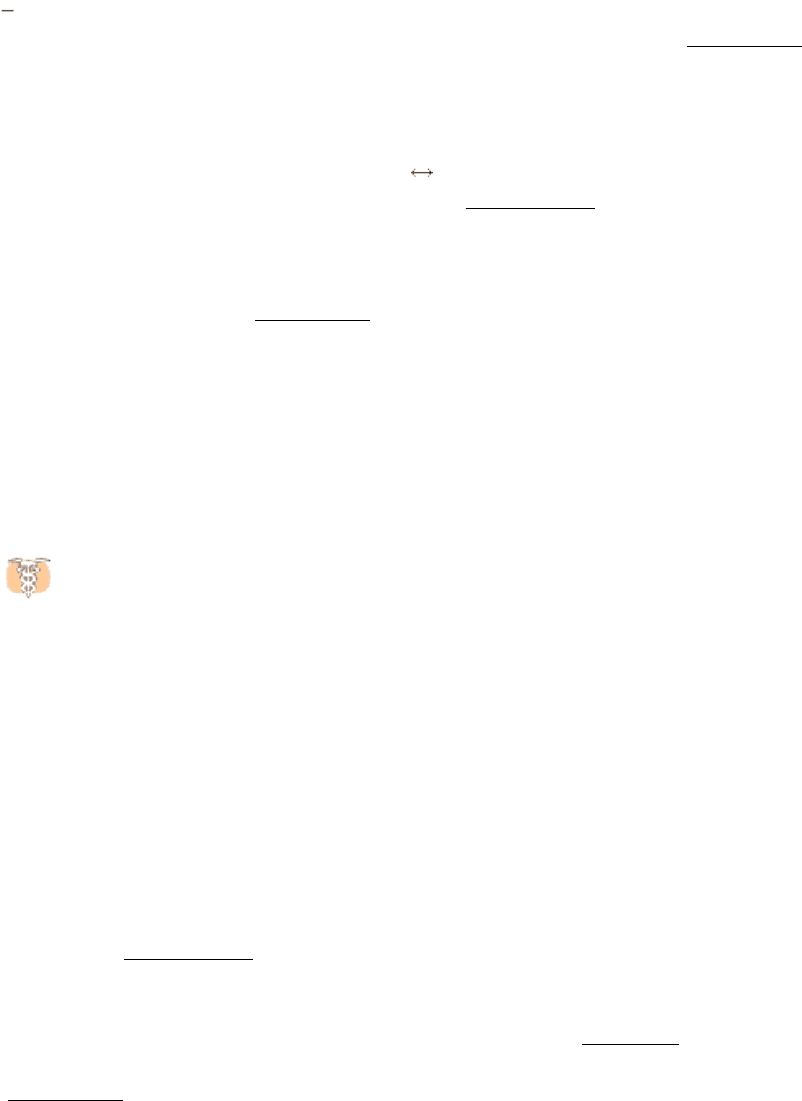
After a meal rich in carbohydrates, blood-glucose levels rise, leading to an increase in glycogen synthesis in the liver.
Although insulin is the primary signal for glycogen synthesis, other, nonhormonal mechanisms also function in the liver.
One signal is the concentration of glucose in the blood, which normally ranges from about 80 to 120 mg per 100 ml (4.4
6.7 mM). The liver senses the concentration of glucose in the blood and takes up or releases glucose accordingly. The
amount of liver phosphorylase a decreases rapidly when glucose is infused (Figure 21.21). After a lag period, the amount
of glycogen synthase a increases, which results in the synthesis of glycogen. In fact, phosphorylase a is the glucose
sensor in liver cells. The binding of glucose to phosphorylase a shifts its allosteric equilibrium from the active R form to
the inactive T form. This conformational change renders the phosphoryl group on serine 14 a substrate for protein
phosphatase 1. It is significant that PP1 binds tightly to phosphorylase a but acts catalytically only when glucose induces
the transition to the T form. Recall that the R T transition of muscle phosphorylase a is unaffected by glucose and is
thus unaffected by the rise in blood-glucose levels (Section 21.2.2).
How does glucose activate glycogen synthase? Phosphorylase b, in contrast with phosphorylase a, does not bind the
phosphatase. Consequently, the conversion of a into b is accompanied by the release of PP1, which is then free to
activate glycogen synthase (Figure 21.22). Removal of the phosphoryl group of inactive glycogen synthase b converts it
into the active a form. Initially, there are about 10 phosphorylase a molecules per molecule of phosphatase. Hence, the
activity of glycogen synthase begins to increase only after most of phosphorylase a is converted into b. This remarkable
glucose-sensing system depends on three key elements: (1) communication between the serine phosphate and the
allosteric site for glucose, (2) the use of PP1 to inactivate phosphorylase and activate glycogen synthase, and (3) the
binding of the phosphatase to phosphorylase a to prevent the premature activation of glycogen synthase.
21.5.4. A Biochemical Understanding of Glycogen-Storage Diseases Is Possible
Edgar von Gierke described the first glycogen-storage disease in 1929. A patient with this disease has a huge
abdomen caused by a massive enlargement of the liver. There is a pronounced hypoglycemia between meals.
Furthermore, the blood-glucose level does not rise on administration of epinephrine and glucagon. An infant with this
glycogen-storage disease may have convulsions because of the low blood-glucose level.
The enzymatic defect in von Gierke disease was elucidated in 1952 by Carl and Gerty Cori. They found that glucose 6-
phosphatase is missing from the liver of a patient with this disease. This was the first demonstration of an inherited
deficiency of a liver enzyme. The liver glycogen is normal in structure but present in abnormally large amounts. The
absence of glucose 6-phosphatase in the liver causes hypoglycemia because glucose cannot be formed from glucose 6-
phosphate. This phosphorylated sugar does not leave the liver, because it cannot cross the plasma membrane. The
presence of excess glucose 6-phosphate triggers an increase in glycolysis in the liver, leading to a high level of lactate
and pyruvate in the blood. Patients who have von Gierke disease also have an increased dependence on fat metabolism.
This disease can also be produced by a mutation in the gene that encodes the glucose 6-phosphate transporter. Recall
that glucose 6-phosphate must be transported into the lumen of the endoplasmic reticulum to be hydrolyzed by
phosphatase (Section 16.3.5). Mutations in the other three essential proteins of this system can likewise lead to von
Gierke disease.
Seven other glycogen-storage diseases have been characterized (Table 21.1). In Pompe disease (type II), lysosomes
become engorged with glycogen because they lack α-1,4-glucosidase, a hydrolytic enzyme confined to these organelles
(Figure 21.23). The Coris elucidated the biochemical defect in another glycogen-storage disease (type III), which cannot
be distinguished from von Gierke disease (type I) by physical examination alone. In type III disease, the structure of liver
and muscle glycogen is abnormal and the amount is markedly increased. Most striking, the outer branches of the
glycogen are very short. Patients having this type lack the debranching enzyme (α-1,6-glucosidase), and so only the
outermost branches of glycogen can be effectively utilized. Thus, only a small fraction of this abnormal glycogen is
functionally active as an accessible store of glucose.
A defect in glycogen metabolism confined to muscle is found in McArdle disease (type V). Muscle phosphorylase
activity is absent, and the patient's capacity to perform strenuous exercise is limited because of painful muscle cramps.
The patient is otherwise normal and well developed. Thus, effective utilization of muscle glycogen is not essential for
life. The results of phosphorus-31 nuclear magnetic resonance studies of these patients have been very informative. The