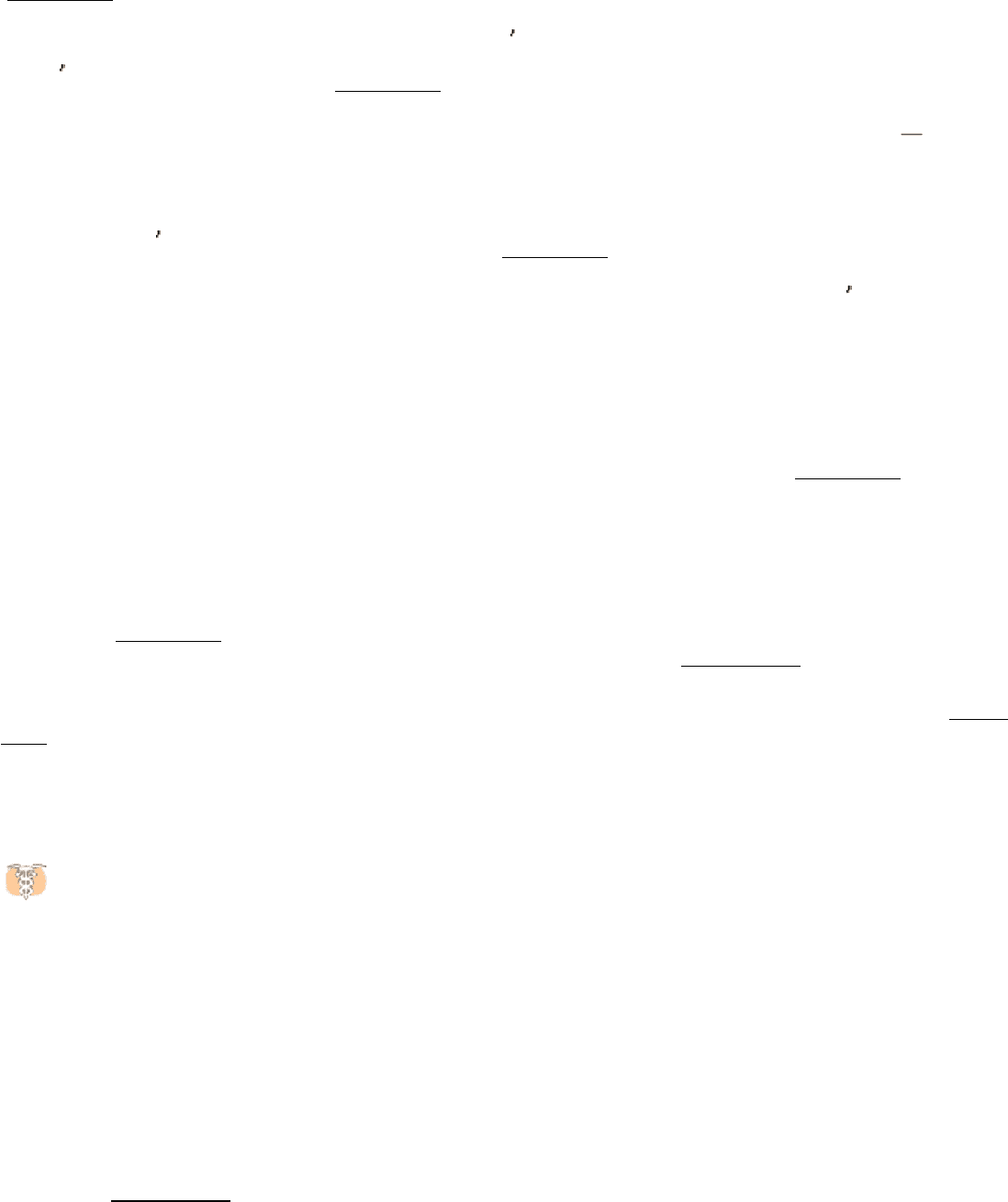
other ligands. In these compounds, the cobalt is in the +3 oxidation state.
The rearrangement reactions catalyzed by coenzyme B
12
are exchanges of two groups attached to adjacent carbon atoms
(Figure 22.13). A hydrogen atom migrates from one carbon atom to the next, and an R group (such as the -CO-S-CoA
group of methylmalonyl CoA) concomitantly moves in the reverse direction. The first step in these intramolecular
rearrangements is the cleavage of the carbon-cobalt bond of 5
-deoxyadenosylcobalamin to form coenzyme B
12
(Co
2+
)
and a 5
-deoxyadenosyl radical, -CH
2
·) (Figure 22.14). In this homolytic cleavage reaction, one electron of the Co-C
bond stays with Co (reducing it from the +3 to the +2 oxidation state) while the other stays with the carbon atom,
generating a free radical. In contrast, nearly all other cleavage reactions in biological systems are heterolytic
an
electron pair is transferred to one of the two atoms that were bonded together.
What is the role of this very unusual -CH
2
· radical? This highly reactive species abstracts a hydrogen atom from the
substrate to form 5
-deoxyadenosine and a substrate radical (Figure 22.15). This substrate radical spontaneously
rearranges: the carbonyl CoA group migrates to the position formerly occupied by H on the neighboring carbon atom to
produce a different radical. This product radical abstracts a hydrogen atom from the methyl group of 5
-deoxyadenosine
to complete the rearrangement and return the deoxyadenosyl unit to the radical form. The role of coenzyme B
12
in such
intramolecular migrations is to serve as a source of free radicals for the abstraction of hydrogen atoms.
An essential property of coenzyme B
12
is the weakness of its cobalt-carbon bond, the facile cleavage of which generates
a radical. To facilitate the cleavage of this bond, enzymes such as methylmalonyl CoA mutase displace the
benzamidazole group from the cobalt and coordinate the cobalamin through a histidine residue (Figure 22.16). The steric
crowding around the cobalt-carbon bond within the corrin ring system contributes to the bond weakness.
22.3.4. Fatty Acids Are Also Oxidized in Peroxisomes
Although most fatty acid oxidation takes place in mitochondria, some oxidation takes place in cellular organelles called
peroxisomes (Figure 22.17). These organelles are characterized by high concentrations of the enzyme catalase, which
catalyzes the dismutation of hydrogen peroxide into water and molecular oxygen (Section 18.3.6). Fatty acid oxidation in
these organelles, which halts at octanyl CoA, may serve to shorten long chains to make them better substrates of β
oxidation in mitochondria. Peroxisomal oxidation differs from β oxidation in the initial dehydrogenation reaction (Figure
22.18). In peroxisomes, a flavoprotein dehydrogenase transfers electrons to O
2
to yield H
2
O
2
instead of capturing the
high-energy electrons as FADH
2
, as occurs in mitochondrial β oxidation. Catalase is needed to convert the hydrogen
peroxide produced in the initial reaction into water and oxygen. Subsequent steps are identical with their mitochondrial
counterparts, although they are carried out by different isoforms of the enzymes.
Zellweger syndrome, which results from the absence of functional peroxisomes, is characterized by liver, kidney,
and muscle abnormalities and usually results in death by age six. The syndrome is caused by a defect in the import
of enzymes into the peroxisomes. Here we see a pathological condition resulting from an inappropriate cellular
distribution of enzymes.
22.3.5. Ketone Bodies Are Formed from Acetyl Coenzyme A When Fat Breakdown
Predominates
The acetyl CoA formed in fatty acid oxidation enters the citric acid cycle only if fat and carbohydrate degradation are
appropriately balanced. The reason is that the entry of acetyl CoA into the citric acid cycle depends on the availability of
oxaloacetate for the formation of citrate, but the concentration of oxaloacetate is lowered if carbohydrate is unavailable
or improperly utilized. Recall that oxaloacetate is normally formed from pyruvate, the product of glycolysis, by pyruvate
carboxylase (Section 16.3.1). This is the molecular basis of the adage that fats burn in the flame of carbohydrates.