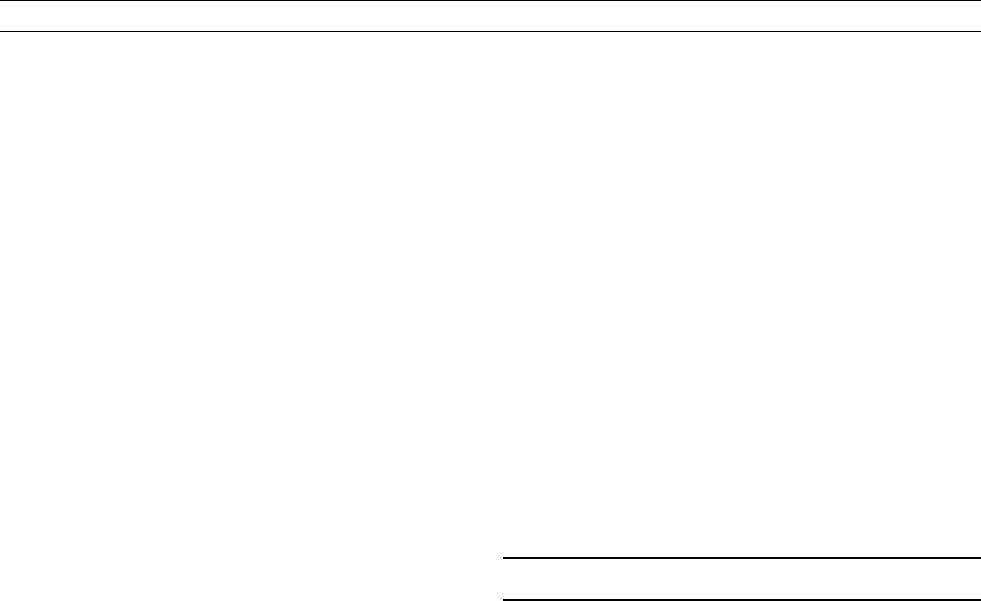
the Stillwater complex, and often the needles are perpendicular to the
plagioclase c-axes. Further support for this interpretation was obtained
from hysteresis loops made along the direction of the principal axes
of the remanence ellipsoids. Single-domain magnetite could also be
responsible for an inverted magnetic fabric in susceptibility anisotropy.
However, the same fabric would be obtained if paramagnetic minerals
are responsible for the AMS. The AMR was used to correct estimates
of paleointensity, which were affected by the preferential alignment of
the magnetite grains.
Ann M. Hirt
Bibliography
Borradaile, G.J., and Henry, B., 1997. Tectonic applications of mag-
netic susceptibility and its anisotropy. Earth-Science Reviews, 42:
49–93.
Cox, A., and Doell, R.R., 1967. Measurement of high-coercivity
magnetic anisotropy. In Collinson, D.W., Creer, K.M., and
Runcorn, S.K. (eds.), Methods in Paleomagnetism. Amsterdam:
Elsevier, pp. 477–482.
de Wall, H., and Worm, H.-U., 1993. Field dependence of magnetic
anisotropy in pyrrhotite: effects of texture and grain shape. Physics
of the Earth and Planetary Interiors, 76: 137–149.
Fuller, M.D., 1960. Anisotropy of susceptibility and the natural rema-
nent magnetization of some Welsh slates. Nature, 186: 790–792.
Girdler, R.W., 1961. The measurement and computation of anisotropy
of magnetic susceptibility of rocks. Geophysical Journal of the
Royal Astronomical Society, 5:34–45.
Graham, J.W., 1954. Magnetic susceptibility anisotropy, an un-
exploited petrofabric element. Bulletin of the Geological Society
of America, 65: 1257–1258.
Hargraves, R.B., 1959. Magnetic anisotropy and remanent magnetiza-
tion in hemo-ilmenite ore deposits at Allard Lake, Quebec. Journal
of Geophysical Research, 64: 1565–1578.
Howell, L.G., Martinez, J.D., and Statham, E.H., 1958. Some observa-
tions on rock magnetism. Geophysics, 23: 285–298.
Jackson, M., 1991. Anisotropy of magnetic remanence: a brief review
of mineralogical sources, physical origins, and geological applica-
tions, and comparison with susceptibility anisotropy. Pure and
Applied Geophysics, 136:1–28.
Jackson, M.J., and Borradaile, G., 1991. On the origin of the magnetic
fabric in purple Cambrian slates of north Wales. Tectonophysics,
194:49–58.
Jackson, M., Gruber, W., Marvin, J., and Banerjee, S.K., 1988. Partial
anhysteretic remanence and its anisotropy: Applications and grain
size-dependence. Geophysical Research Letters, 15: 440–443.
Jelinek, V., 1996. Theory and measurement of the anisotropy of iso-
thermal remanent magnetization of rocks. Travaux Géophysique,
37: 124–
134.
McCabe, C., Jackson, M., and Ellwood, B.B., 1985. Magnetic aniso-
tropy in the Trenton limestone: results of a new technique,
anisotropy of anhysteretic susceptibility. Geophysical Research
Letters, 12: 333–336.
Nye, J.F., 1957. Physical Properties of Crystals. London: Oxford
University Press.
Rochette, P., Jackson, M.J., and Aubourg, C., 1992. Rock magnetism
and the interpretation of anisotropy of magnetic susceptibility.
Reviews of Geophysics, 30: 209–226.
Selkin, P.A., Gee, J.S., Tauxe, L., Meurer, W.P., and Newell, A.J.,
2000. The effect of remanence anisotropy on paleointensity esti-
mates: a case study from the Archean Stillwater Complex. Earth
and Planetary Science Letters, 183: 403–416.
Stacey, F.D., and Banerjee, S.K., 1974. The Physical Principles of Rock
Magnetism. Amsterdam: Elsevier Scientific Publishing Company.
Stephenson, A., Sadikun, S., and Potter, D.K., 1986. A theoretical
and experimental comparison of the anisotropies of magnetic
susceptibility and remanence in rocks and minerals. Geophysical
Journal of the Royal Astronomical Society, 84: 185–200.
Tauxe, L., Constable, C., Stokking, L., and Badgley, C., 1990. Use of
anisotropy to determine the origin of characteristic remanent mag-
netization in the Siwalik red beds of northern Pakistan. Journal of
Geophysical Research—Solid Earth and Planets, 95: 4391–4404.
Tan, X.D., and Kodama, K.P., 2002. Magnetic anisotropy and paleo-
magnetic inclination shallowing in red beds: evidence from the
Mississippian Mauch Chunk Formation, Pennsylvania. Journal of
Geophysical Research—Solid Earth, 107B11):2311, doi: 10.1029/
2001JB001636.
Tarling, D.H., and Hrouda, F., 1993. The Magnetic Anisotropy of
Rocks. London: Chapman & Hall.
Tauxe, L., 2002. Paleomagnetic Principles and Practice. Dordrecht:
Kluwer Academic Press.
Cross-references
Magnetic Susceptibility, Anisotropy
Magnetization, Anhysteretic Remanent (ARM)
Magnetization, Isothermal Remanent (IRM)
Magnetization, Natural Remanent (NRM)
Magnetization, Thermoremanent (TRM)
MAGNETIC SHIELDING
There are numerous uses for magnetic shields in research and consumer
electronics. In geophysical research, magnetic shields are used in
paleomagnetic laboratories to protect samples and improve the perfor-
mance of sample magnetometers. Research shields range in size from
10 cm to 15 m.
The irony in magnetic shielding theory is that a shield actually adds
another magnetic field. This new field is specifically designed to be
equal and opposite to the existing field, resulting in a nullification
of, not a removal of the ambient magnetic fields. This is analogous
to gravitational forces at Lagrangian points, or the balance of centripe-
tal and gravitational forces on orbiting bodies, or noise cancellation by
antiphase headphones.
Materials used
Diverse materials and techniques are used for magnetic shielding. The
choice depends on the kind of fields to be opposed (e.g., AC vs DC)
and the specific application. In general, shielding can be accomplished
by using one of two physical phenomenon, either electrical conductiv-
ity or strong magnetic responses. Electrical conductors carry currents
that produce a magnetic field perpendicular to the current direction.
To generate these currents in a conductive sheet, there must be an ori-
ginal, varying magnetic field. Within the conductor, these currents pro-
duce a new magnetic field in the opposite direction to this exciting
field ( Lenz’s law). By surrounding a site with a good conductor, the
volume will be shielded from varying fields coming from all direc-
tions. The important parameters for efficient shielding by conductors
are: conductivity and thickness of the shielding material, frequency
of the magnetic field, and the completeness of enclosure. Of course,
electrical wires can be powered externally to produce a magnetic field
of almost any configuration, including the nullification case of shield-
ing. This will be considered in detail later.
Materials with strong magnetic properties, usually metals containing
Fe, Ni, or Co, can react to changing magnetic fields by generating an
oppositely directed field (magnetic permeability) or can be tuned to
oppose a steady field (magnetic remanence). Efficient shielding by mag-
netic materials depends on the material, its treatment history, as well
as the ambient field characteristics of frequency, strength, gradient,
and source direction.
540 MAGNETIC SHIELDING