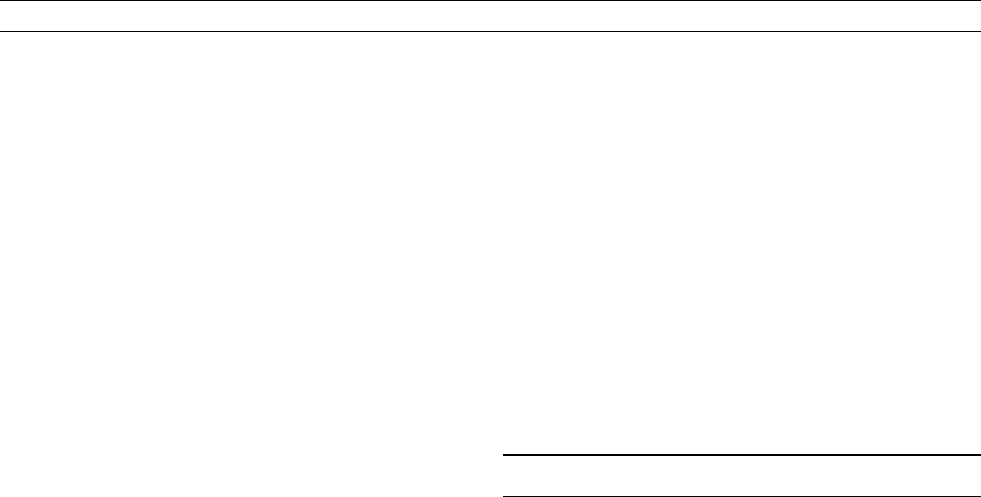
Cottrell, R.D., and Tarduno, J.A., 2000. In search of high fidelity geo-
magnetic paleointensities: A comparison of single crystal and
whole rock Thellier-Thellier analyses. Journal of Geophysical
Research, 105: 23579–23594.
Cox, A., 1968. Lengths of geomagnetic polarity intervals. Journal of
Geophysical Research, 73: 3247–3260.
Davis, K.E., 1981. Magnetite rods in plagioclase as the primary carrier
of stable NRM in ocean floor gabbros. Earth and Planetary
Science Letters, 55: 190–198.
Dunlop, D.J., and Özdemir, Ö., 1997. Rock Magnetism, Fundamentals
and Frontiers, Cambridge: Cambridge University Press, 573 pp.
Glatzmaier, G.A., Coe, R.S., Hongre, L., and Roberts, P.H., 1999. The
role of the Earth’s mantle in controlling the frequency of geomag-
netic reversals. Nature, 401: 885–890.
Goguitchaichvili, A., Alva-Valdivia, L.M., Urrutia, J., and Morales, J.,
2002. On the reliability of Mesozoic Dipole Low: New absolute
paleointensity results from Paraná flood basalts (Brazil). Geophysi-
cal Research Letters, 29: 10.1029/2002GL0152 42.
Halls, H.C., and Zhang, B.X., 1998. Uplift structure of the southern
Kapuskasing zone from 2.45 Ga dike swarm displacement. Geol-
ogy, 26:67–70.
Heller, R., Merrill, R.T., and McFadden, P.L., 2002. The variation of
intensity of earth’s magnetic field with time. Physics of the Earth
and Planetary Interiors, 131: 237–249.
Juarez, T., Tauxe, L., Gee, J.S., and Pick, T., 1998. The intensity of the
Earth’s magnetic field over the past 160 million years. Nature, 394:
878–881.
Kosterov, A.A., Prévot, M., and Perrin, M., 1997. Paleointensity of the
Earth’s magnetic field in the Jurassic: New results from a Thellier
study of the Lesotho Basalt, southern Africa. Journal of Geophysi-
cal Research, 102: 24859–24872.
McElhinny, M.W., and Larson, R.L., 2003. Jurassic dipole low defined
from land and sea data. Eos, Transactions of the American Geo-
physical Union, 84: 362–366.
Özdemir, Ö., and Dunlop, D.J., 1985. An experimental study of che-
mical remanent magnetizations of synthetic monodomain titanoma-
ghemites with initial thermoremanent magnetizations. Journal of
Geophysical Research., 90: 11513–11523.
Prévot, M., Derder, M.E., McWilliams, M.O., and Thompson, J.,
1990. Intensity of the Earth’s magnetic field: Evidence for a
Mesozoic dipole low. Earth and Planetary Science Letters, 97:
129–139.
Smirnov, A.V., and Tarduno, J.A., 2003. Magnetic hysteresis monitor-
ing of Cretaceous submarine basaltic glass during Thellier paleoin-
tensity experiments: Evidence for alteration and attendant low field
bias. Earth and Planetary Science Letters, 206: 571–585.
Smirnov, A.V., Tarduno, J.A., and Pisakin, B.N., 2003. Paleointensity
of the early geodynamo (2.45 Ga) as recorded in Karelia: a single
crystal approach. Geology, 31: 415–418.
Smirnov, A.V., and Tarduno, J.A., 2004. Secular variation of the Late
Archean-Early Proterozoic geodynamo. Geophysical Research
Letters, 31, L16607.
Tarduno, J.A., Cottrell, R.D., and Smirnov, A.V., 2001. High geomag-
netic field intensity during the mid-Cretaceous from Thellier ana-
lyses of single plagioclase crystals. Science, 291: 1779–1783.
Tarduno, J.A., Cottrell, R.D., and Smirnov, A.V., 2002. The Cre-
taceous Superchron Geodynamo: Observations near the tangent
cylinder. Proceedings of the National Academy of Sciences. 99:
14020–14025.
Tarduno, J.A., and Smirnov, A.V., 2004. The paradox of low field
values and the long-term history of the geodynamo. In Channell,
J.E.T., Kent, D.V., Lowrie, W., and Meert, J.G., (eds.), Timescales
of the Paleomagnetic Field. American Geophysical Union Geo-
physical Monograph Series 145. Washington, DC: American
Geophysical Union.
Tauxe, L., and Staudigel, H., 2004. Strength of the geomagnetic field
in the Cretaceous Normal Superchron: New data from submarine
basaltic glass of the Troodos Ophiolite. Geochemistry, Geophysics
and Geosystems, 5, Q02H06.
Thellier, E., and Thellier, O., 1959. Sur l’intensité du champ magné-
tique terrestre dans le passé historique et géologique. Annales Geo-
physicae, 15: 285–376.
Valet, J.P., 2003. Time variations in geomagnetic intensity. Reviews of
Geophysics, 41: 1004.
Verwey, E.J.W., 1939. Electronic conduction of magnetite (Fe
3
O
4
) and
its transition point at low-temperature. Nature, 44: 327–328.
Cross-references
Geodynamo, Numerical Simulations
Geomagnetic Dipole Field
Inner Core
Magnetization, Thermoremanent (TRM)
Paleomagnetism
PALEOINTENSITY, ABSOLUTE, TECHNIQUES
Introduction
The unique possibility to extract the ancient field intensity from mag-
netic remanence of rocks is to duplicate the acquisition of magnetiza-
tion by laboratory experiments in presence of a field with a known
intensity. This requires a precise knowledge of the mechanisms that
govern the magnetization, which is actually the major difficulty inher-
ent to the paleointensity experiments. Acquisition of remanence by
strong magnetic fields does not concern natural rocks because the geo-
magnetic field has a weak intensity (unless they are affected by light-
ning but this has no interest for paleomagnetism). Another way of
magnetizing rocks is by thermal activation, which leads to rotation
of some magnetic moments in the direction of the field. The natural
magnetization relevant from this process is called a thermoremanent
magnetization (TRM). Thermoremanence is acquired by most igneous
rocks during cooling from above the Curie temperature (T
c
) in the pre-
sence of the Earth’s magnetic field. Magnetic relaxation, in which
remanent magnetization of an assemblage of magnetic grains decays
with time, is the most straightforward effect of thermal activation. As
temperature decreases through a critical temperature called “blocking
temperature” (T
b
), an individual magnetic grain experiences a dramatic
increase in relaxation time, which will continue down to room tem-
perature. Thus the orientation of the magnetic moment can remain
stable at the surface temperature and it will be resistant to effects of
magnetic fields after original cooling. Depending on specific para-
meters inherent to the grain, this magnetic memory can be preserved
for billions of years. Rock specimens contain many magnetic grains
that can have different characteristics (size, shape etc.) and are thus
frequently associated with a distribution of blocking temperatures T
b
)
from a few tens of degrees up to T
c
of the magnetic mineral carrying
the magnetization.
Thellier-Thellier technique and derivatives
A very specific characteristic of the total TRM is that it can be sepa-
rated into the successive magnetizations that were acquired in distinct
temperature intervals. For example, TRM of an igneous rock contain-
ing magnetite can be broken into portions acquired within successive
windows of blocking temperatures from its Curie temperature (T
c
)of
580
C down to room temperature. The portion of TRM blocked in
any particular T
b
window is referred to as “partial TRM, ” abbreviated
pTRM. The total TRM is the vector sum of the pTRMs contributed by
all blocking temperature windows. The Individual pTRMs depend
only on the magnetic field during cooling through their respective T
b
intervals and are not affected by magnetic fields applied during
PALEOINTENSITY, ABSOLUTE, TECHNIQUES 753