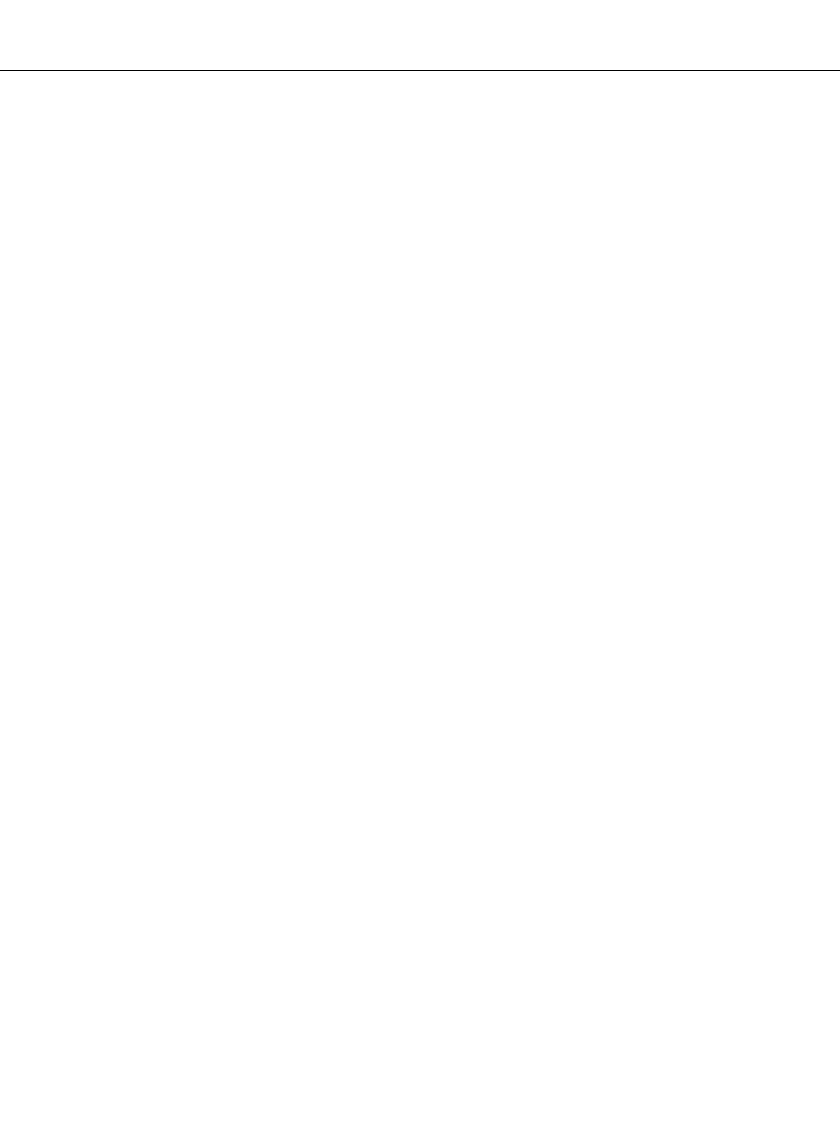
VALVE REGULATED LEAD-ACID BATTERIES 24.27
24.5 CHARGING CHARACTERISTICS
24.5.1 General Considerations
15
Charging the VRLA battery, like charging other secondary systems, is a matter of replacing
the energy depleted during discharge. Because this process is inefficient, it is necessary to
return more than 100% of the energy removed during discharge. The amount of energy
necessary for recharge depends on how deeply the battery was discharged, the method of
recharge, the recharge time, and the temperature. The overcharge required in the lead-acid
battery is associated with the generation of gases and corrosion of the positive-grid materials.
In conventional flooded lead-acid batteries the gases generated are released from the system,
resulting in a loss of water, which is replenished by maintenance. The VRLA battery incor-
porates the gas recombination principle which allows the oxygen generated at normal over-
charge rates to be reduced at the negative plate, eliminating oxygen outgassing. Hydrogen
gas generation has been substantially reduced by the use of nonantimonial lead grid material.
The corrosion of the positive grid has been reduced by the use of pure or special alloy lead.
Also, the effects of corrosion of the positive grid have been minimized by the element
construction.
Charging can be accomplished by various methods. Constant-voltage charging is the con-
ventional method for lead-acid batteries and is also preferred for VRLA batteries. However,
constant current, taper current, and variations thereof can also be used.
VRLA batteries during cycling and float charge can become unbalanced in that the cor-
rosion rate of the positive becomes unequal to the self-discharge rate of the negative. A
suggested remedy
16
is to utilize a catalyst in the vent space of the cell to restore balance by
recombining hydrogen and oxygen.
24.5.2 Constant-Voltage Charging
Constant-voltage charging is the most efficient and fastest method of charging a VRLA
battery. Figure 24.33 shows the recharge times at various charge voltages for a cell discharged
to 100% of capacity. The charger required to achieve these times at given voltages must be
capable of at least the 2C rate. If the constant-voltage charger used has less than the 2C rate
of charge capability, the charge times should be lengthened by the hourly rate at which the
charger is limited; that is, if the charger is limited to the C /10 rate, then 10 h should be
added to each of the charge voltage-time relationships; if the charger is limited to the C/5
rate, then 5 h should be added, and so on. There are no limitations on the maximum current
imposed by the charging characteristics of the battery.
Figure 24.34 is a set of curves of charge current versus time for 2.5-Ah batteries charged
by a constant voltage of 2.45 V with chargers limited to 2-, 1-, and 0.3-A currents. As
shown, the only difference in these three charges is the length of time necessary to recharge
the battery.
Figure 24.35a shows the charge rate versus time and the percent of previous discharge
capacity returned versus time at 2.35-V constant voltage for a battery discharged at the
C/ 2.5 rate to 80% DOD. The time necessary to recharge the battery to 100% of the previous
discharge capacity is 1.5 h. The charger used was capable of an output in the 4C range and
of voltage regulation at the terminals of better than 0.1%. The initial high inrush of current
caused internal cell heating, which enhanced charge acceptance. If the battery had been more
deeply discharged, the length of time to reach 100% capacity would have increased. The
current decays exponentially with time, and after 3 h on charge, the current has decayed to
a low level. At 2.35 V, the cell will accept whatever current is necessary to maintain capacity.
Figure 24.35b is a similar plot but for a battery charged at 2.50 V constant voltage. All
of the capacity taken out on the previous discharge is returned in approximately one-half
hour.