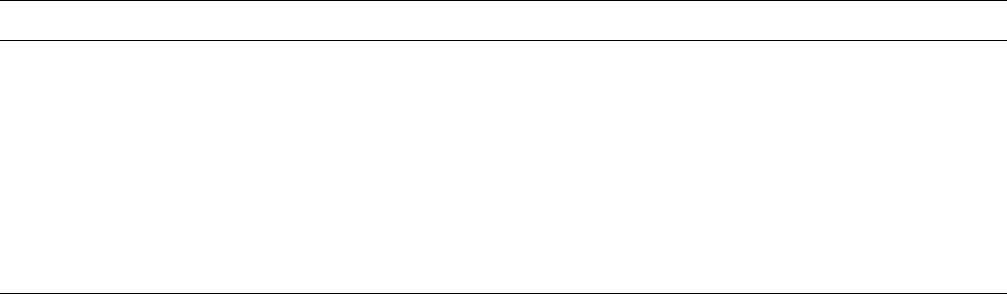
(vinylcinnamate) doped with a NLO chromophore. In this
guest–host system it was possible to achieve a 20% loading
level of guest molecules without phase separation. Although
the T
g
of the system is found to be dependent on the chro-
mophore concentration, plasticization of the chromophore
was remarkably small. Absorption measurements indicate
that the polar order in the poled film persists for a long
period of time (many months) without any change [35].
Table 49.4 shows d
33
values obtained from poled films of
some side-chain polymers [36–43].
Also, the Langmuir–Blodgett (LB) technique has been
employed to prepare oriented thin films of organic polymers
for NLO investigations. An LB film containing 20 bilayers
of alternating monolayer of two different polymers yield a
thickness of 60 nm and a w
(2)
zzz
of 11:2 10
16
esu cm per
bilayer at 1:047 mm [44]. Molecular nonlinearity in this LB
film is supposed be arising from charge-transfer excitation
in the polymer with the sulfonyl group as the acceptor and
amino group as the donor. Optical waveguides capable of
guiding blue light with low loss (2---6 dB cm
1
) over several
centimeters have been fabricated from NLO polymers using
the LB technique. However, it was necessary to use another
NLO inactive polymer (t-butyl methacrylate polymer) as
buffer layer in order to prevent the formation of inversion
center in the film [45–48]. An LB film containing 168
bilayers (thickness 0:44 m m) showed waveguide attenu-
ation at 457.9 nm of 5.8 dB/cm for TE polarization and
2.6 dB/cm for TM polarization. The SHG measurements
on a 0.88-mm-thick LB film results in a w
(2)
zzz
value of
8 10
9
esu. Similar w
(2)
zzz
values (d
33
¼ 5:3 10
9
esu)
have been obtained for Langmuir–Schaefer films of meso-
genic moieties containing polysilane copolymers [49].
Current strategies for developing highly w
(2)
activities of
NLO polymers basically involve molecular design concepts,
same as presented in the previous sections. The key steps
are: (i) design of high mb chromophores; (ii) improvement
of temporal stability of NLO response in polymer matrices;
and (iii) poling efficiency of the polymeric systems [50].
Among these, the most important factor might be to design
highly efficient NLO chromophores. Therefore, to under-
stand the structure–property relationship of chromophores
with high w
(2)
values, quantum mechanical analysis based on
a simple two-level model description and bond-order alter-
nation principle have been investigated [51,52]. Table 49.5
shows values of mb at the off-resonance wavelength of
1907 nm for some representative chromophores, with im-
proved optical nonlinearity [53,54]. A large enhancement of
optical nonlinearity can be obtained by extending the length
of p-bridged chains with strong electron acceptor such as
the cyano group. It was shown that very large electro-optic
(E-O) coefficients could be achieved by introducing highly
efficient chromophores into amorphous polymer matrix
such as poly(methyl methaacrylate)(PMMA), polycarbon-
ate, polyquinoline, etc. [55–59]. In the case of PMMA added
with 17.5% of chromophore 10, the E-O coefficient was
reported to be r
33
¼ 105 pmV
1
at 1:33 mm [60]. This is
more than three times higher than that of lithium niobate
(LiNbO
3
; r
33
¼ 31 pmV
1
), which has been used as a ma-
terial for E-O modulators.
To improve the temporal and the thermal stabilities of
poled NLO dipoles, polyurethanes can be considered as one
of the best matrix materials, because of their extensive
formation of H-bonding between the urethane linkages
which would increase the rigidity of the polymer chain.
Several researchers succeeded in making greatly enhanced
NLO polymeric systems by using polyurethane backbone
[61–68]. Also, polyimides [69–80], polyetherimides
[81,82], polyamides [83–89], and polyesters [90–94] as
matrix polymers can also provide an enhanced thermal
stability of aligned dipoles due to high glass transition
temperature (T
g
) characteristics which also result in the
chain stiffness. By incorporating the NLO chromophores
into these polymer backbones, much improved long-term
thermal stability of NLO activity were obtained.
Another promising approach for making efficient NLO
systems is the employment of dendritic polymers [95–111].
Compared to common polymers, dendritic NLO polymers
can provide many advantages from the viewpoint of
structural variation, optimization of E-O values, thermal
stability, optical loss, etc. Jen’s group prepared a multiarm
TABLE 49.4. The NLO coefficient d
33
( ¼ 0:5x
(2)
333
) of poled main-chain NLO polymers.
Polymer d
33
(pm/V) l(mm) References
Polyurea 6.6 1.064 [29]
BisA-NAT 90 1.064 [35]
BisA-NAT 30 1.064 [35]
BisA-NPDA 13.5 1.064 [36]
d
31
¼ 3
Polyurethane 40 1.064 [37]
NLO chromophore tethered 120 1.064 [38]
Polyimide (NLO chromophore tethered) 4.6–5.5 1.064 [39]
NLO-chromophore-tethered acrylate 60 [40]
Polycinnamate: photocross-linked with
NLO chromophore
<11.5–21.5 1.54 [41,42]
NONLINEAR OPTICAL PROPERTIES OF POLYMERS / 803