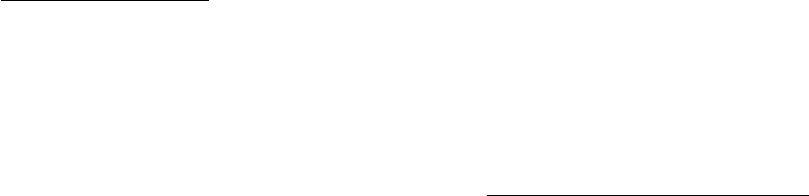
have repose times between eruptive episodes of as
much as 10
5
10
6
y. Thus, ignimbrite sequences are
built of multiple depositional flow units; each unit rep-
resents one explosive event. These ignimbrites may be
separated by surge and fall deposits (Figures 10.29 and
10.37).
A cooling unit is a pyroclastic flow deposit nearly in-
stantaneously laid down that cools as a thermal entity.
A simple cooling unit can comprise one depositional
unit, or it can be made of two or more that are em-
placed nearly simultaneously so that there are no inter-
nal cooling breaks, such as intervening less welded tuff
(discussed later). Discerning boundaries between de-
positional units within a simple cooling unit can be
challenging; compositional discontinuities and inter-
vening surge and fall deposits can be helpful. A com-
pound cooling unit consists of a succession of flows
emplaced closely in time so that only partial cooling
breaks occur between depositional units.
Composition of Deposits. Most ignimbrites are rhyo-
lite; fewer are dacite, trachyte, and phonolite; andesite
is uncommon. Juvenile pyroclasts are predominantly
ash-size vitroclasts and lesser crystals. Larger cognate
pumice lapilli are typical, whereas lithic lapilli can be
conspicuous in some deposits. Crystals include euhe-
dral to subhedral intact phenocrysts formed in the
preeruption magma chamber and phenoclasts of the
same ancestory but broken during eruption. These
primary crystals may be very sparse, 1% of the de-
posit, but can range to as much as about 50%. Some
lithic clasts are cognate crystalline fragments related
to the erupted magma, such as from the crystallized
wall of the magma chamber. Accidental lithic frag-
ments (xenoliths) can be chunks of rock torn from the
enlarging conduit during explosive eruption, wallrock
from the preeruption magma chamber, and loose rock
fragments on the ground picked up and incorporated
into a turbulent flow. The proportion of lithic frag-
ments, pumice clasts, and phenocrysts in the deposit
can vary widely, each from 0% to as much as 50% or
so, whereas juvenile vitric ash particles are always in
abundance.
Compositionally zoned ignimbrites were first well
documented by Lipman et al. (1966; see also Hildreth,
1981). In some of these ash-flow deposits zonation is
cryptic and can only be discerned by laboratory analy-
ses, whereas in others it is quite conspicuous in the
field (Figure 10.38a). Normally, the proportion of phe-
nocrysts in the rock, as well as their sizes, increase
stratigraphically upward in the sheet, as do FeO, MgO,
and CaO, whereas the lower part of the ignimbrite
sheet has a more evolved composition. A common
zonation is a basal, high-silica rhyolite overlain by low-
silica rhyolite, by dacite, or, in instances of strong zon-
ing, by andesite. In some deposits, lateral zonation is
also evident from proximal parts near the source to dis-
tal parts tens of kilometers distant. Strong vertical and
horizontal zonation in an ash-flow deposit may make it
difficult to correlate isolated exposures of one deposi-
tional unit. Other useful correlation tools include pre-
cise isotopic dating and paleomagnetic analyses (Best
et al., 1995).
Zoned ignimbrite deposits are derived by more or
less systematic withdrawal from preeruption magma
chambers that have compositional gradients (Figure
10.38b). The more evolved top of the chamber is
erupted first; then successively deeper parts are
erupted, producing, in the deposit, an inverted zona-
tion of that in the chamber. It is obvious that a sample
of bulk tuff in most instances does not accurately rep-
resent any of the preeruption magma; the tuff sample
is an explosive mixture of pyroclasts derived from dif-
ferent compositional zones in the chamber. Moreover,
the tuff has likely suffered some differential loss of fine
vitric ash by elutriation from the ash flow. Unaltered
pumice lumps hosted in the tuff, on the other hand,
represent unmodified samples of the preeruption
magma. Therefore, analyses of pumice best portray
compositional variations in the preeruption magma
body. Likewise, analyses of single glass shards, usually
by electron microprobe, accurately reflect the melt
composition.
Secondary Zonation after Deposition. Ash-flow de-
posits commonly have other zonal features that are su-
perimposed on any primary compositional zonation
just described. Secondary processes formed during
cooling of the hot mass of pyroclasts and entrapped gas
include (Figure 10.39):
1. Welding and compaction
2. Vapor-phase crystallization of minerals from the en-
trapped gas
3. Devitrification (delayed crystallization) of the glassy
material
Welding is the bonding of hot glass particles. Be-
cause of the weight of overlying pyroclasts on these
soft sticky particles, they are compacted together and
trapped gas is squeezed out, collapsing pore spaces and
vesicles within pumice fragments and reducing the
bulk porosity of the tuff. In some deposits, interstitial
gas that is largely steam may be partially resorbed into
vitroclasts, reducing their viscosity and, hence, pro-
moting welding more or less independently of com-
paction (Sparks et al., 1999). But generally the most in-
tense welding and compaction occur in the lower
portion of the flow, though not at the base, where faster
cooling prevents much welding (Figure 10.39). Weld-
ing and compaction operate simultaneously to create
the typical eutaxitic fabric of welded ash-flow tuffs, in
which pumice lapilli and glass shards are flattened into
Magma Extrusion: Field Relations of Volcanic Rock Bodies
273