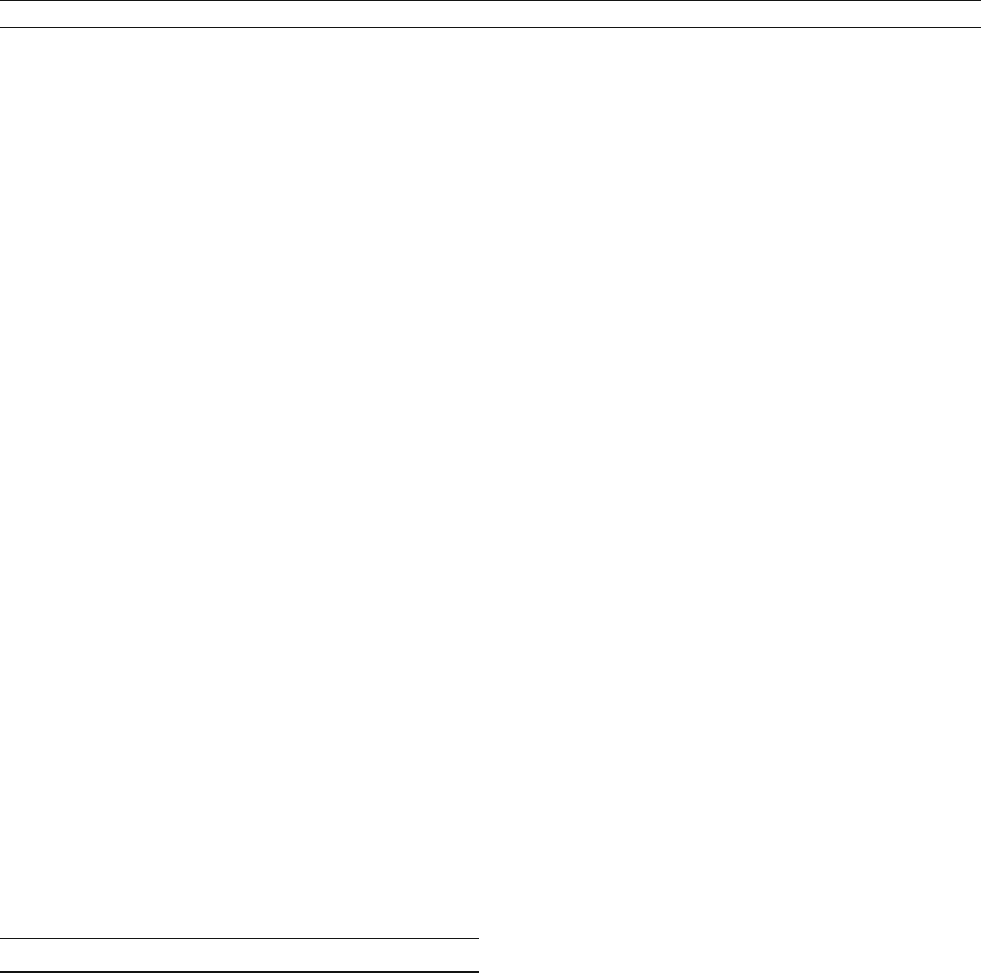
Bibliography
Arkhipov, S.A., Ehlers, J., Johnson, R.G. and Wright, H.E. Jr., 1995. Gla-
cial drainage towards the Mediterranean during the Middle and Late
Pleistocene. Boreas, 24, 196–206.
Atwater, B.F., 1986. Pleistocene Glacial-lake Deposits of the Sanpoil River
Valley Northeastern Washington. U.S. Geological Survey Bulletin
1661, 39pp.
Baker, V.R., and Bunker, R.C., 1985. Cataclysmic late Pleistocene flooding
from glacial Lake Missoula: A review. Quaternary Sci. Rev., 4,1–41.
Baker, V.R., Benito, G., and Rudoy, A.N., 1993. Paleohydrology of late
Pleistocene superflooding, Altai Mountains, Siberia. Science, 259,
348–350.
Bjorck, S., 1995. A review of the history of the Baltic Sea, 13.0–8.0 ka BP.
Quaternary Int., 27,19–40.
Broecker, W.S. and Denton, G.H., 1989. The role of ocean-atmosphere
reorganizations in glacial cycles. Geochim. Cosmochim. Acta, 53,
2465–2501.
Clarke, G., Leverington, D., Teller, J., and Dyke, A., 2003. Superlakes,
megafloods, and abrupt climate change. Science, 301, 922–923.
Grosswald, M.G., 1980. Late Weichselian Ice Sheet of northern Eurasia.
Quaternary Res., 13,1–32.
Leverington, D., Mann, J.D., and Teller, J.T., 2002. Changes in the bathy-
metry and volume of glacial Lake Agassiz between 9,200 and
7,700
14
C yr B.P. Quaternary Res., 57, 244–252.
Mangerud, J., Astakhov, V., Jakobsson, M., and Svendsen, J.I., 2001.
J. Quaternary Res., 16, 773–777.
O’Connor, J.E., 1993. Hydrology, hydraulics and sediment transport of
Pleistocene lake Bonneville flooding on the Snake River, Idaho. Geolo-
gical Society of America Special Paper 274, 83pp.
Ryan, W.B.F., Major, C.O., Lericolais, G., and Goldstein, S.L., 2003. Cat-
astrophic flooding of the Black Sea. Annu. Rev. Earth Planet. Sci., 31,
525–554.
Teller, J.T., Leverington, D.W., and Mann, J.D., 2002. Freshwater outbursts
to the oceans from glacial Lake Agassiz and their role in climate change
during the last deglaciation. Quaternary Sci. Rev., 21, 879–887.
Cross-references
Cordilleran Ice Sheet
Last Glacial Termination
Late Quaternary Megafloods
Laurentide Ice Sheet
Proglacial Lacustrine Sediments
Quaternary Climate Transitions and Cycles
Scandinavian Ice Sheet
The 8,200-year BP Event
Thermohaline Circulation
Younger Dryas
GLACIAL SEDIMENTS
Glacial sediments are formed in association with glacier ice in
subglacial, ice marginal, lacustrine and marine environments.
In such diverse environments, sediment can be reworked and
deposited by a very wide range of processes, including subglacial
lodgement, deformation and melt-out, subaerial and subaqueous
mass-movements, fluvial processes, and settling through a water
column. Numerous attempts have been made to classify the
resulting range of sediments in schemes of greater or lesser com-
plexity (Dreimanis, 1989), incorporating many varieties of “till”
(e.g., flow till, lowered till, waterlain till, iceberg till). Many of
these proposed sediment types are difficult to distinguish in the
field, however, and a simple classification scheme is now favored
by most workers. A fundamental distinction can be made between
primary glaciogenic deposits, formed by exclusively glacial pro-
cesses (such as subglacial lodgement, melt-out and deformation),
and secondary deposits, formed by the reworking of glaciogenic
debris by processes not unique to glacial environments (Lawson,
1989). In terms of their internal characteristics, secondary glacio-
genic deposits can be indistinguishable from sediments formed
by similar processes in non-glacial environments and so, seen in
isolation, they do not provide unequivocal evidence for the former
presence of glacier ice. A glacial origin, however, can be deter-
mined from the context of such sediment facies, particularly the
range of associated facies and structures, and the form and distri-
bution of overlying landforms. It is therefore helpful to take a hier-
archical approach to the analysis and interpretation of glacial
sediments, in which individual facies are interpreted in terms of
their process of deposition, and sediment-landform associations
(comprising suites of genetically-related facies) are used to recon-
struct former depositional environments (cf. Walker, 1992; Benn
and Evans, 1998).
The characteristics of glacial sediments reflect the processes
of entrainment, transport, and deposition experienced by debris
as it travels through a glaciated basin. One of the most distinc-
tive characteristics of glacial sediments is the presence of erra-
tics, or exotic, far-traveled material. For Quaternary deposits,
the provenance of erratics can often be pinpointed, allowing
patterns of glacier flow to be reconstructed with some accuracy.
For older successions, however, this may not be possible, espe-
cially if sediment exposure or preservation is poor. Erratics do
not occur in all glacial deposits, however, and some are dominated
by local material,eroded and redeposited close to its source. Trans-
port processes can also impart a distinctive glacial signature
on sediments. Debris transported at glacier beds is commonly
subjected to high inter-particle contact forces, and the resulting
abrasion and crushing of particles produces distinctive clast
morphologies and particle size distributions. Clasts are commonly
striated (particularlyfiner lithologies)with smooth, polished facets
on their upper and lower surfaces, and may have asymmetrical
stoss-lee or bullet-shaped forms. The presence of such clasts in a
deposit does not necessarily mean, however, that it was deposited
in a subglacial environment, because glacial debris can be carried
far beyond glacier margins by various processes prior to final
deposition. Some of these processes (such as fluvial transport) will
result in rapid clast rounding and obliteration of striae and other
glacial signatures, whereas others (such as iceberg rafting) may
carry debris for hundreds of kilometers with little or no modifica-
tion. Particle size distributions of subglacially-transported debris
commonly exhibit a very broad range of grain sizes, from clay or
siltup to pebbles or larger, and are typically bimodal or polymodal.
The finer particles (<63 mm) are formed during subglacial abra-
sion, and are sometimes known as rock flour. Other particle size
modes may reflect fracturing or crushing of larger clasts along
joints, grain boundaries and other weaknesses. Whereas subglacial
transport produces distinct debris characteristics, debris trans-
ported in englacial and supraglacial positions generally undergoes
little or no modification and commonly retains the character of the
parent debris. For this reason, supraglacial and englacial debris
transport is known as passive transport, in distinction to active
subglacial transport (Boulton, 1978; Benn and Evans, 1998),
although some limited clast modification can occur during passive
transport. Passive transport is particularly important in mountain
environments, where large quantities of debris are delivered to gla-
cier surfaces by rockfall and other mass movement processes. Flu-
vial processes can also transport significant quantities of sediment
through some glaciers, resulting in significant clast rounding and
size sorting. Thus, although striated, faceted clasts and rock flour
are typical of subglacially transported debris, they are not essential
382 GLACIAL SEDIMENTS