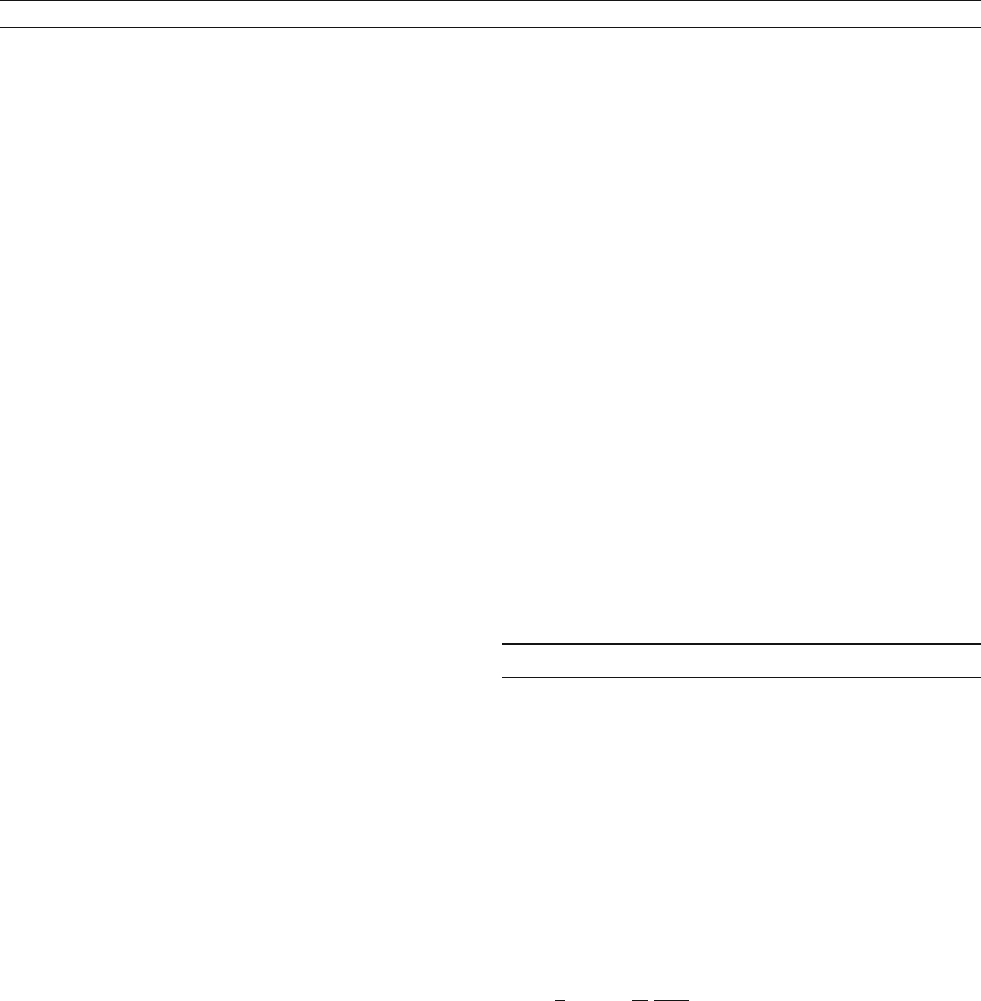
pollen is used to support the ecological hypothesis that these
mountains provided long-term refugia for the temperate taxa
(Tzedakis, 1993).
Increased detail is apparent in the pollen analysis conducted
on a borehole called Ioannina 284 (Figure P85) (Tzedakis et al.,
2002, 2003). The mean sampling interval has been increased to
approximately 225 years. Because of the increased temporal
resolution of the record, greater detail within a single glacial
or interglacial becomes evident. The onset of the last intergla-
cial has been defined by the authors as occurring when tree pol-
len consistently rises above 50% frequency, and when tree
pollen concentration exceeds 200,000 grains per cm
3
; the onset
of the ensuing colder stadial occurs when tree pollen con-
sistently drops below 50%. Thus, the Last Interglacial is rep-
resented in the interval from 96.7 to 83.4 cm depth, or
approximately 127.3–111.7 kyr ago (Tzedakis et al., 2003).
After the chronology has been determined, the pollen changes
in Ioannina 284 are compared with other climatic data
from the North Atlantic region. The data indicate that the
millennial-scale expansions and contractions of tree popula-
tions may be in phase with climate events in the North Atlantic
region and Greenland regions. However, sufficient moisture to
maintain refugial temperate tree populations is proposed to be
linked to the topographic variability of the Pindus Mountains
(Tzedakis et al., 2002).
The pollen analyses from these two sites show how both
paleoclimatological and paleoecological interpretations may
be extracted from the studies. Furthermore, the means of chron-
ological control will vary with the age of the sediments. With
basins that encompass the Holocene epoch, laminated sedi-
ments may allow high temporal resolution. For older basins
(including marine sediments), age may be based on biostrati-
graphic correlations. Regionally significant climate changes
usually cannot be derived from a single pollen record: several
fossil pollen records together from a user-defined region are
necessary in order to confirm that patterns in pollen spectrum
have significance beyond the single site examined.
Margaret Kneller
Bibliography
Bradley, R.S., 1999. Paleoclimatology, Reconstructing Climates of the
Quaternary. Burlington, MA: Harcourt/Academic Press, 613pp.
Davis, M., 1969. Climatic changes in southern Connecticut recorded by
pollen deposition at Rogers Lake. Ecology, 50, 409–422.
Davis, O.K., 2002. Late Neogene environmental history of the Northern
Bonneville Basin: A review of palynological studies. In Hershler, R.,
Madsen, D.B., and Currey, D.R. (eds.), Great Basin Aquatic Systems
History. Washington, D.C.: Smithsonian Institution, pp. 295–307.
Davis, O.K., and Moutoux, T.E., 1998. Tertiary and Quaternary vegetation
history of the Great Salt Lake, Utah, USA. J. Paleolimnol., 19,
417–427.
Deevey, E.S., 1969. Coaxing history to conduct experiments. Bioscience,
19,40–43.
Erdtman, G., 1943. An Introduction to Pollen Analysis. Waltham, MA:
Chronica Botanica Company, 239pp.
Fægri, K., and Iversen, J., 1975. Textbook of Pollen Analysis, third revised
edition. New York: Hafner Press, 295pp.
Fægri, K., Kaland, P.E., and Krzywinski, K., 1989. Textbook of Pollen Ana-
lysis, by Knut Fægri and Johs. Iversen. Caldwell, NJ: The Blackburn
Press, 328pp.
McAndrews, J.H., Berti, B., and Norris, G., 1973. Key to the Quaternary
Pollen and Spores of the Great Lakes Region. Toronto, ON: The Royal
Ontario Museum, 64pp.
Pennisi, E., 2003. Modernizing the Tree of Life. Science, 300, 1692–1697.
Roberts, N., 1998. The Holocene: An Environmental History, 2nd edn.
Oxford, UK: Blackwell Publishers Ltd, 316pp.
Traverse, A., 1988. Paleopalynology. Winchester, MA: Allen & Unwin
Inc., 600pp.
Twell, D., 2000. Pollen: Structure, Development and Function. In Nature
Encyclopedia of Life Sciences. London, UK: Nature Publishing Group.
http://80-www.els.net.osiyou.cc.columbia.edu:2048 /[doi:10.1038 /npg.
els.0002039].
Tzedakis, P.C., 1993. Long-term tree populations in northwest Greece
through multiple Quaternary climatic cycles. Nature, 364, 437–440.
Tzedakis, P.C., Lawson, I.T., Frogley, M.R., Hewitt, G.M., and Preece, R.C.,
2002. Buffered tree population changes in a Quaternary refugium:
Evolutionary implications. Science, 297, 2044–2047.
Tzedakis, P.C., Frogley, M.R., and Heaton, T.H.E., 2003. Late Interglacial
conditions in southern Europe: Evidence from Ioannina, northwest
Greece. Glob Planet. Change, 367, 157–170.
von Post, L., 1967 [1916]. Forest tree pollen in south Swedish peat bog
deposits, a translation by M.B. Davis and K. Fægri of Om skogstrad-
spollen i sydsvenska torfmosselagerfoljder (foredragsreferat) (Geolo-
giska Foereningen i Skockholm. Foerhandlingar 38:384), with an
introduction by Knut Fægri and Johs. Iversen. Pollen et Spores,
9, 378–401.
Cross-references
Dating, Biostratigraphic Methods
Late Quaternary-Holocene Vegetation Modeling
Paleobotany
Palynology
Quaternary Vegetation Distribution
POTASSIUM-ARGON/ARGON-ARGON DATING
General principl es
The K-Ar dating method utilizes the decay of the naturally-
occurring radioactive isotope of potassium,
40
K, into an isotope
of the noble gas, argon (i.e.,
40
Ar).
40
K decays into two different
daughter products: the most common path (89.5%) is via beta
decay into
40
Ca; the remainder of the decay paths arrive at
40
Ar, mostly via electron capture, but also rarely via positive
beta (positron emission) decay. The total decay half life for
40
K is about 1.25 billion years, which means that there is still
some of the isotope remaining from the formation of the solar
system. Because of the branching decay scheme, the standard
radiometric age equation needs to be modified as follows:
t ¼
1
l
ln 1 þ
l
l
e
40
Ar
40
K
ð1Þ
where l
e
is the decay constant leading to
40
Ar (0.581 10
10
yr
1
) and l is the total decay constant for both branches
(5.543 10
10
yr
1
).
Much of the natural radioactivity surrounding us is due to
40
K, but fortunately, it is a quite rare isotope, only accounting
for about one atom in 8,570 atoms of potassium. Despite the
rarity of
40
K and the fact that only about one atom in ten decays
into
40
Ar, the K-Ar system is arguably the most versatile of the
long-lived decay scheme dating methods. It is nearly an ideal
chronometer because K is a major element present in almost
all rocks and minerals, but the act of mineral formation is
usually exceptionally efficient at excluding atmospheric argon
from the mineral’s crystal structure. Radiogenic
40
Ar is well
POTASSIUM-ARGON/ ARGON-ARGON DATING 823