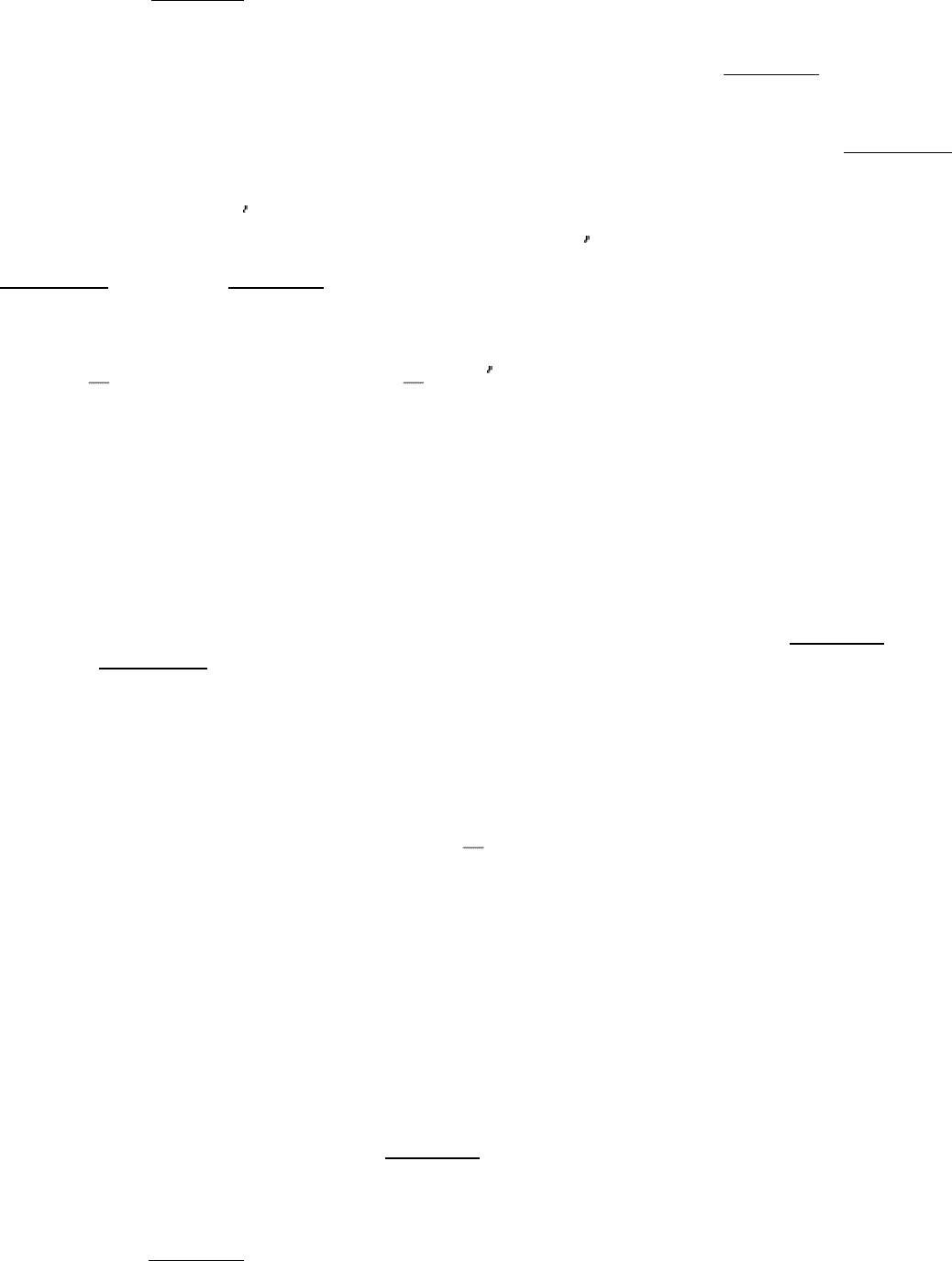
How can mammalian DNA be cloned and expressed by E. coli? Recall that most mammalian genes are mosaics of
introns and exons (Section 5.6). These interrupted genes cannot be expressed by bacteria, which lack the machinery to
splice introns out of the primary transcript. However, this difficulty can be circumvented by causing bacteria to take up
recombinant DNA that is complementary to mRNA. For example, proinsulin, a precursor of insulin, is synthesized by
bacteria harboring plasmids that contain DNA complementary to mRNA for proinsulin (Figure 6.23). Indeed, bacteria
produce much of the insulin used today by millions of diabetics.
The key to forming complementary DNA (cDNA) is the enzyme reverse transcriptase. As discussed in Section 5.3.1, a
retrovirus uses this enzyme to form a DNA-RNA hybrid in replicating its genomic RNA. Reverse transcriptase
synthesizes a DNA strand complementary to an RNA template if it is provided with a DNA primer that is base-paired to
the RNA and contains a free 3
-OH group. We can use a simple sequence of linked thymidine [oligo(T)] residues as the
primer. This oligo(T) sequence pairs with the poly(A) sequence at the 3
end of most eukaryotic mRNA molecules
(Section 5.4.4), as shown in Figure 6.24. The reverse transcriptase then synthesizes the rest of the cDNA strand in the
presence of the four deoxyribonucleoside triphosphates. The RNA strand of this RNA-DNA hybrid is subsequently
hydrolyzed by raising the pH. Unlike RNA, DNA is resistant to alkaline hydrolysis. The single-stranded DNA is
converted into double-stranded DNA by creating another primer site. The enzyme terminal transferase adds
nucleotides
for instance, several residues of dG to the 3 end of DNA. Oligo(dC) can bind to dG residues and prime
the synthesis of the second DNA strand. Synthetic linkers can be added to this double-helical DNA for ligation to a
suitable vector. Complementary DNA for all mRNA that a cell contains can be made, inserted into vectors, and then
inserted into bacteria. Such a collection is called a cDNA library.
Complementary DNA molecules can be inserted into vectors that favor their efficient expression in hosts such as E. coli.
Such plasmids or phages are called expression vectors. To maximize transcription, the cDNA is inserted into the vector
in the correct reading frame near a strong bacterial promoter site. In addition, these vectors ensure efficient translation by
encoding a ribosome-binding site on the mRNA near the initiation codon. Clones of cDNA can be screened on the basis
of their capacity to direct the synthesis of a foreign protein in bacteria. A radioactive antibody specific for the protein of
interest can be used to identify colonies of bacteria that harbor the corresponding cDNA vector (Figure 6.25). As
described in Section 6.2.3, spots of bacteria on a replica plate are lysed to release proteins, which bind to an applied
nitrocellulose filter. A
125
I-labeled antibody specific for the protein of interest is added, and autoradiography reveals the
location of the desired colonies on the master plate. This immunochemical screening approach can be used whenever a
protein is expressed and corresponding antibody is available.
6.3.2. Gene-Expression Levels Can Be Comprehensively Examined
Most genes are present in the same quantity in every cell
namely, one copy per haploid cell or two copies per diploid
cell. However, the level at which a gene is expressed, as indicated by mRNA quantities, can vary widely, ranging from
no expression to hundreds of mRNA copies per cell. Gene-expression patterns vary from cell type to cell type,
distinguishing, for example, a muscle cell from a nerve cell. Even within the same cell, gene-expression levels may vary
as the cell responds to changes in physiological circumstances.
Using our knowledge of complete genome sequences, it is now possible to analyze the pattern and level of expression of
all genes in a particular cell or tissue. One of the most powerful methods developed to date for this purpose is based on
hybridization. High-density arrays of oligonucleotides, called DNA microarrays or gene chips, can be constructed either
through lightdirected chemical synthesis carried out with photolithographic microfabrication techniques used in the
semiconductor industry or by placing very small dots of oligonucleotides or cDNAs on a solid support such as a
microscope slide. Fluorescently labeled cDNA is hybridized to the chip to reveal the expression level for each gene,
identifiable by its known location on the chip. (Figure 6.26).
The intensity of the fluorescent spot on the chip reveals the extent of transcription of a particular gene. DNA chips have
been prepared that contain oligonucleotides complementary to all known open reading frames, 6200 in number, within
the yeast genome (Figure 6.27). An analysis of mRNA pools with the use of these chips revealed, for example, that