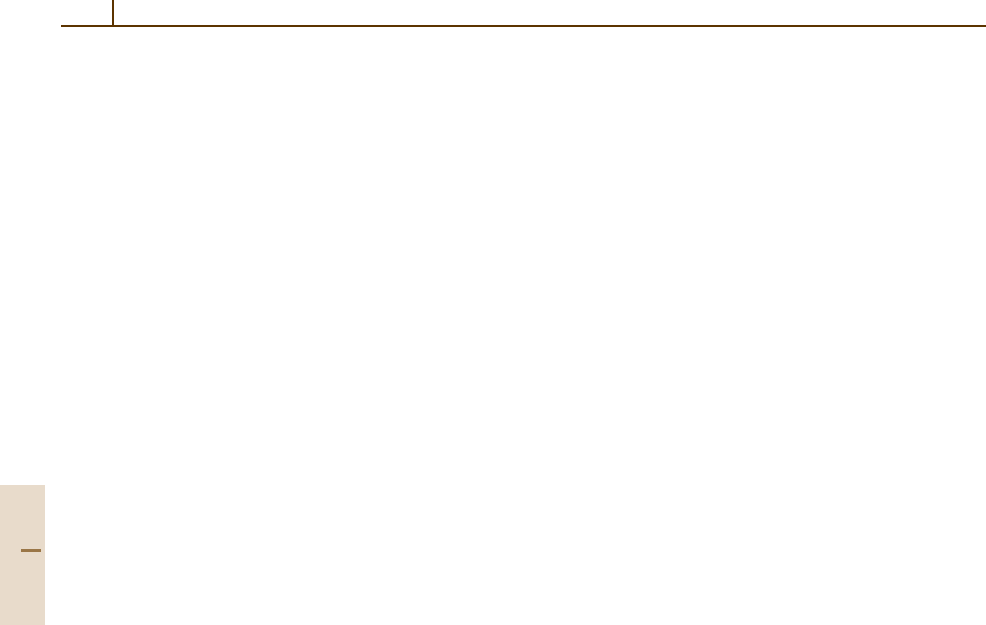
556 Part 3 Classes of Materials
Viscosity
As explained in the introduction, glasses pass through
three viscosity ranges between the melting temperature
and room temperature: the melting range, the super-
cooled melt range, and the solidification range. The
viscosity increases during the cooling of the melt,
starting from 10
0
–10
4
dPa s. A transition from a li-
quid to a plastic state is observed between 10
4
and
10
13
dPa s.
The softening point, i. e. the temperature where
the viscosity is 10
7.6
dPa s, identifies the plastic range
in which glass parts rapidly deform under their own
weight. The glass structure can be described as solidi-
fied or “frozen” above 10
13
dPa s. At this viscosity, the
internal stresses in glass anneal out equalize in approx-
imately 15 min. The temperature at which the viscosity
is 10
13
dPa s is called the upper annealing point, and is
important for the annealing of glasses.
In accordance with ISO 7884-8, the rate of change of
the relativelinear thermal expansioncan be used to deter-
mine the transformation temperature T
g
, which is close
to the temperature at which the viscosity is 10
13
dPa s.
Precision optical surfaces may deform and refractive
indices may change if a temperature of T
g
−200 K is
exceeded during any thermal treatment.
Coefficient of Linear Thermal Expansion
The typical curve of the linear thermal expan-
sion of a glass begins with an increase in slope
from absolute zero to approximately room tempera-
ture. Then a nearly linear increase to the beginning
of the plastic behavior follows. The transforma-
tion range is distinguished by a distinct bending
of the expansion curve, which results from the
increasing structural rearrangement in the glass.
Above this range, the expansion again exhibits
a nearly linear increase, but with a noticeably greater
slope.
Two averaged coefficients of linear thermal expan-
sion α are usually given: α
30/70
, averaged from −30
◦
C
to +70
◦
C, which is the relevant value for room tempera-
ture; and α
20/300
, averaged from +20
◦
Cto+300
◦
C,
which is the standard international value. These values
are listed in Table 3.4-16.
3.4.5.4 Thermal Properties
Thermal Conductivity
The range of values for the thermal conductivity of
glasses at room temperature extends from 1.38 W/mK
(pure vitreous silica) to about 0.5W/mK (high-lead-
content glasses). The most commonly used silicate
glasses have values between 0.9and1.2W/mK. All
data in Table 3.4-16c are given for a temperature of
90
◦
C, with an accuracy of ±5%.
Specific Thermal Capacity
The mean isobaric specific heat capacities c
p
(20
◦
C;
100
◦
C) listed in Table 3.4-16c were measured from
the heat transfer from a hot glass sample at 100
◦
C
into a liquid calorimeter at 20
◦
C. The values of
c
p
(20
◦
C; 100
◦
C) and also of the true thermal cap-
acity c
p
(20
◦
C) for silicate glasses range from 0.42 to
0.84 J/gK.
3.4.6 Vitreous Silica
Vitreous silica has a unique set of properties. It is pro-
duced either from natural quartz by fusion or, if extreme
purity is required, by chemical vapor deposition or via
a sol–gel routes. Depending on the manufacturing pro-
cess, variable quantities impurities are incorporated in
the ppm or ppb range, such as Fe, Mg, Al, Mn, Ti,
Ce, OH, Cl, and F. These impurities and radiation-
induced defects, as well as complexes of impurities and
defects, and also overtones, control the UV and IR trans-
mittance. In the visible part of the spectrum, Rayleigh
scattering from thermodynamically caused density fluc-
tuations dominates. Defects are also responsible for
the damage threshold under radiation load, and for
fluorescence. The refractive index n and the absorption
constant K as a function of wavelength are found in
Fig. 3.4-29.
The highest transmittance is required for applica-
tions in optical communication networks, in optics for
lithography, and in high-power laser physics. For cer-
tain applications, for example to increase the refractive
index in the IR in fiber optics, the silica is “doped”
with GeO
2
,P
2
O
5
,B
2
O
3
, etc. in the range of 5–10%.
In such cases the scattering loss increases owing to
concentration fluctuations.
There are also many technical applications which
make use of the chemical inertness, light weight, high
temperature stability, thermal-shock resistance, and low
thermal expansion of vitreous silica. A very low thermal
Part 3 4.6