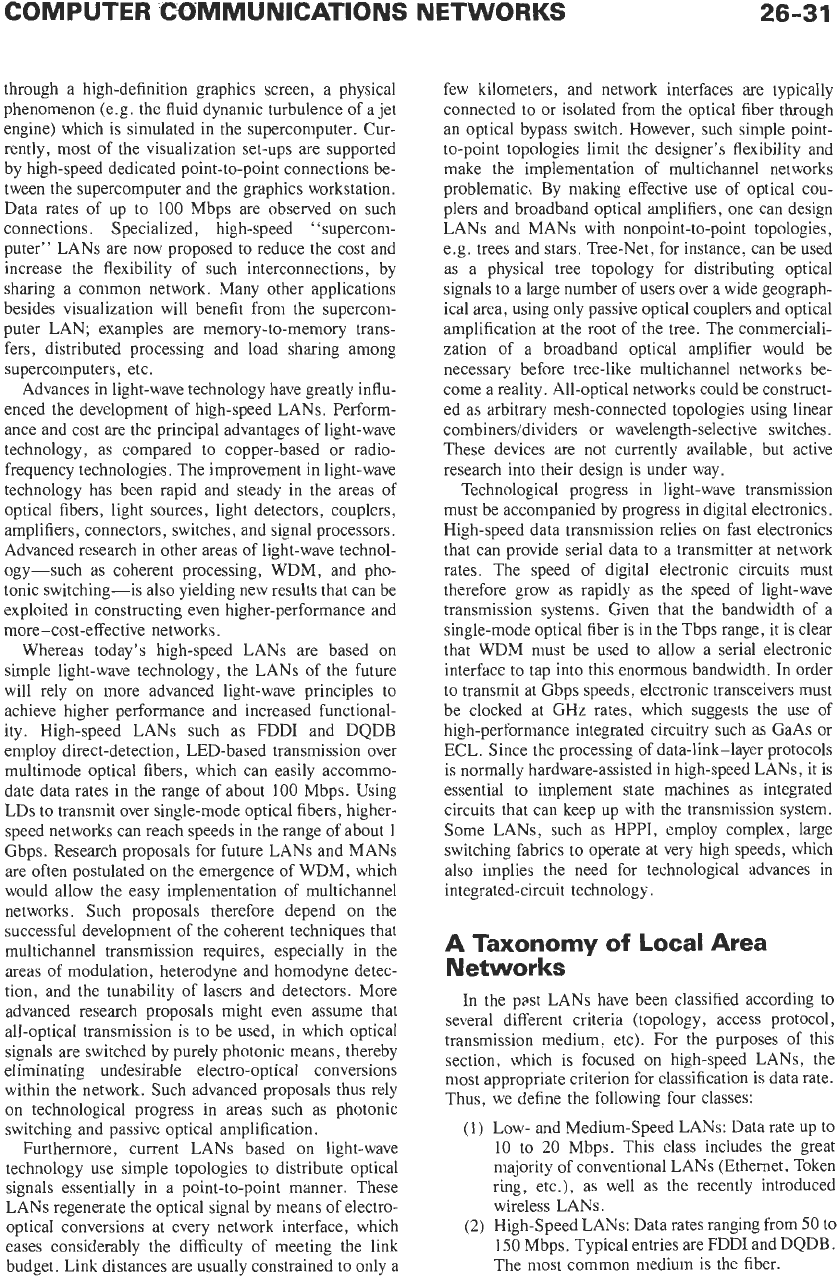
COMPUTER COMMUNICATIONS
through a high-definition graphics screen, a physical
phenomenon (e.g. the fluid dynamic turbulence of a jet
engine) which is simulated in the supercomputer. Cur-
rently, most of the visualization set-ups are supported
by high-speed dedicated point-to-point connections be-
tween the supercomputer and the graphics workstation.
Data rates of up to 100 Mbps are observed on such
connections. Specialized, high-speed “supercom-
puter” LANs are now proposed to reduce the cost and
increase the flexibility of such interconnections, by
sharing a common network. Many other applications
besides visualization will benefit from the supercom-
puter LAN; examples are memory-to-memory trans-
fers, distributed processing and load sharing among
supercomputers, etc.
Advances in light-wave technology have greatly influ-
enced the development of high-speed LANs. Perform-
ance and cost are the principal advantages of light-wave
technology, as compared to copper-based or radio-
frequency technologies. The improvement in light-wave
technology has been rapid and steady in the areas of
optical fibers, light sources, light detectors, couplers,
amplifiers, connectors, switches, and signal processors.
Advanced research in other areas of light-wave technol-
ogy-such as coherent processing, WDM, and pho-
tonic switching-is also yielding new results that can be
exploited in constructing even higher-performance and
more-cost-effective networks.
Whereas today’s high-speed LANs are based on
simple light-wave technology, the LANs of the future
will rely on more advanced light-wave principles to
achieve higher performance and increased functional-
ity. High-speed LANs such as FDDI and DQDB
employ direct-detection, LED-based transmission over
multimode optical fibers, which can easily accommo-
date data rates in the range of about
100
Mbps. Using
LDs
to
transmit over single-mode optical fibers, higher-
speed networks can reach speeds in the range
of
about
1
Gbps. Research proposals for future LANs and MANs
are often postulated on the emergence of WDM, which
would allow the easy implementation of multichannel
networks. Such proposals therefore depend on the
successful development of the coherent techniques that
multichannel transmission requires, especially in the
areas of modulation, heterodyne and homodyne detec-
tion, and the tunability of lasers and detectors. More
advanced research proposals might even assume that
all-optical transmission
is
to
be used, in which optical
signals are switched by purely photonic means, thereby
eIiminating undesirable electro-optical conversions
within the network. Such advanced proposals thus rely
on technological progress in areas such as photonic
switching and passive optical amplification.
Furthermore, current LANs based on light-wave
technology use simple topologies to distribute optical
signals essentially in a point-to-point manner. These
LANs regenerate the optical signal by means of electro-
optical conversions at every network interface, which
eases considerably the difficulty of meeting the link
budget. Link distances are usually constrained to only a
few kilometers, and network interfaces are typically
connected to or isolated from the optical fiber through
an optical bypass switch. However, such simple point-
to-point topologies limit the designer’s flexibility and
make the implementation of multichannel networks
problematic.. By making effective use of optical cou-
plers and broadband optical amplifiers, one can design
LANs and MANs with nonpoint-to-point topologies,
e.g. trees and stars. Tree-Net, for instance, can be used
as a physical tree topology for distributing optical
signals to a large number of users over a wide geograph-
ical area, using only passive optical couplers and optical
amplification at the root of the tree. The commerciali-
zation of a broadband optical amplifier would be
necessary before tree-like multichannel networks be-
come a reality. All-optical networks could be construct-
ed as arbitrary mesh-connected topologies using linear
combinersidividers or wavelength-selective switches.
These devices are not currently available, but active
research into their design is under way.
Technological progress in light-wave transmission
must be accompanied by progress in digital electronics.
High-speed data transmission relies on fast electronics
that can provide serial data to a transmitter at network
rates. The speed of digital electronic circuits must
therefore grow as rapidly as the speed of light-wave
transmission systems. Given that the bandwidth of a
single-mode optical fiber is in the Tbps range, it is clear
that WDM must be used to allow a serial electronic
interface to tap into this enormous bandwidth. In order
to transmit at Gbps speeds, electronic transceivers must
be clocked at GHz rates, which suggests the use of
high-performance integrated circuitry such as GaAs or
ECL. Since the processing of data-link-layer protocols
is normally hardware-assisted in high-speed LANs, it is
essential to implement state machines as integrated
circuits that can keep up with the transmission system.
Some LANs, such as HPPI, employ complex, large
switching fabrics to operate at very high speeds, which
also implies the need for technological advances in
integrated-circuit technology.
A
Taxonomy
of
Local Area
Networks
In the past LANs have been classified according to
several different criteria (topology, access protocol,
transmission medium, etc). For the purposes
of
this
section, which is focused on high-speed LANs, the
most appropriate criterion for classification is data rate.
..
we define the following four classes:
Low- and Medium-Speed LANs: Data rate up to
10
to
20
Mbps. This class includes the great
majority of conventional LANs (Ethernet, Token
ring, etc.), as well as the recently introduced
wireless LANs.
High-speed LANs: Data rates ranging from
50
to
150
Mbps. Typical entries are FDDI and DQDB.
The most common medium is the fiber.