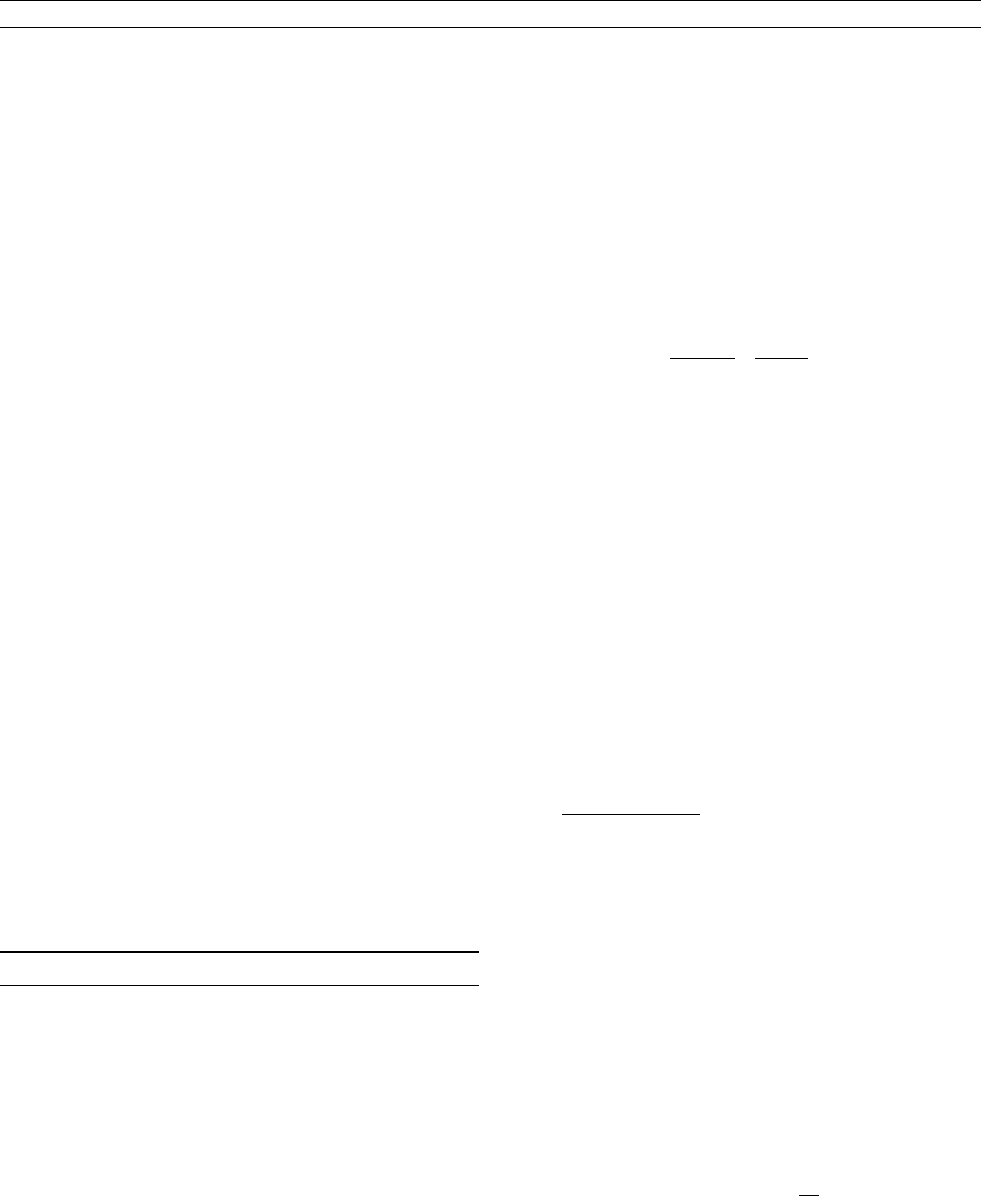
Lasaga, A.C., and Ohmoto, H., 2002. The oxygen geochemical cycle:
Dynamics and Stabilty. Geochim. Cosmochim. Acta, 66, 361–381.
McArthur, J.M., 1994. Recent trends in strontium isotope stratigraphy.
Terra Nova, 6, 331–358.
McConnaughey, T., 1990a.
13
C and
18
O isotopic disequilibrium in biologi-
cal carbonates: 1. Patterns. Geochimica et Cosmochimica Acta, 53,
151–162.
McConnaughey, T., 1990b.
13
C and
18
O isotopic disequilibrium in biologi-
cal carbonates: 2. In vitro simulation of kinetic isotope effects. Geochi-
micia et Cosmochimica Acta, 53, 163–171.
Rothman, D.H., Hayes, J.M., and Summons, R., 2003. Dynamics of the
Neoproterozoic carbon cycle. Proceedings of the National Academy of
Sciences, 100, 8124–8129.
Sackett, W.M., 1989. Stable carbon isotope studies on organic matter in the
marine environment. In Fritz, P., and Fontes, J.C. (eds.), Handbook of
Environmental Isotope Geochemistry. Amsterdam: Elsevier, pp. 139–169.
Schidlowski, M., Hayes, J.M., and Kaplan, I.R., 1983. Isotopic inferences
of ancient biochemistries: Carbon, sulfur, hydrogen, and nitrogen. In
Schopf, J.W. (ed.), Earth’s Earliest Biosphere: Its Origin and Evolu-
tion. Princeton: Princeton University Press, pp. 149–187.
Shaviv, N.J., and Veizer, J., 2003. Celestial driver of Phanerozoic climate?
GSA Today, 13 / 7,4–10.
Shields, G., and Veizer, J., 2002. Precambrian marine carbonate isotope
database: Version 1.1. Geochem. Geophys. Geosyst., 3/6, 12pp.
Veizer, J., 1983. Trace element and isotopes in sedimentary carbonates.
Rev. Mineral., 11, 265–300.
Veizer, J., 1988. The earth and its life: Systems perspective. Origins of Life,
18,13–39.
Veizer, J., 1992. Depositional and diagenetic history of limestones: stable
and radiogenic isotopes. In Clauer, N., and Chaudhuri, S. (eds.), Isoto-
pic signatures in Sedimentary Records. Heidelberg: Springer, pp.
13–48.
Veizer, J., Ala, D., Azmy, K., Bruckschen, P., Buhl, D., Bruhn, F., Carden, G.
A. F., Diener, A., Ebneth, S., Goddéris, Y., Jasper, T., Korte, C.,
Pawellek, F., Podlaha, O.G., and Strauss, H., 1999.
87
Sr/
86
Sr, d
13
Cand
d
18
O evolution of Phanerozoic seawater. Chem. Geol., 161,59–88.
Veizer, J., Goddéris, Y., and François, L.M., 2000. Evidence for decoupling
of atmospheric CO
2
and global climate during the Phanerozoic eon.
Nature, 408, 698–701.
Cross-references
Atmospheric Evolution, Earth
Carbon Cycle
Carbon Dioxide, Dissolved (Ocean)
Carbon Isotopes, Stable
Isotope Fractionation
Marine Carbon Geochemistry
CARBON ISOTOPES, STABLE
General
Carbon has two stable (non-radioactive) isotopes. In nature, the
12
C isotope comprises 98.89% of all carbon and
13
C makes up
the remaining 1.11%. A variety of physico-chemical processes
whose rates are mass-dependent, such as kinetic reactions
involving diffusion, and temperature-controlled equilibrium
reactions serve to “fractionate” the isotopes into proportions
slightly different from the bulk averages. These isotopes are
therefore most useful when used in tandem as isotope ratios
(
13
C/
12
C) in order to explore paleoclimates and paleoenviron-
ments. Carbon isotopes are preserved and expressed in the bulk
composition of a wide variety of materials such as shells, spe-
leothems, bones, leaves, peat, soils, sediments, wood, and food,
and more recently in the composition of specific compounds or
biomarkers contained therein. In some cases, the climate influ-
ence may be quite direct, but in many others the isotopic varia-
tions are less direct, linked to climate effects on the global
carbon cycle and photosynthetic pathways of plants.
Notation
The
13
C/
12
C in materials is measured with a stable-isotope ratio
mass spectrometer (IRMS), taking advantage of the mass dif-
ferences in ionized
13
CO
2
molecules (or
13
CO molecules) rela-
tive to
12
CO
2
(or
12
CO) that cause them to follow different
trajectories in a magnetic field on the path to detectors. The iso-
topic ratio of an unknown sample is ultimately expressed in
permil (%) with the delta (d) notation:
d
13
C ðin % Þ¼
13
C
12
C
sample
13
C
12
C
PDB
1
1000 ð1Þ
where the conventional standard is PDB calcite from belemnite
fossils of the Cretaceous PeeDee Formation of South Carolina.
Although no original PDB standard remains from its initial adop-
tion about five decades ago, there exist secondary standards
such as NBS-21 graphite National Bureau of Standards-21) and
V-PDB (Vienna PDB), whose isotopic composition is known
with respect to PDB (Coplen, 1996). The
13
C/
12
C ratio in PDB
is 0.0112372, so positive d
13
C values have more
13
C (i.e.,
are “enriched”), and negative d
13
C values have less
13
C (i.e., are
“depleted”). More generally when comparing d
13
C between sam-
ples, those with less negative (or more positive) d
13
C values are
more enriched in
13
C relative to samples with more negative (less
positive) d
13
C. The range of d
13
C in many types of materials may
be several permil but some, like ocean water dissolved inorganic
carbon, are very homogeneous ( Figure C19).
When examining carbon isotopic behavior of plants, discri-
mination (D) is often a useful parameter that eliminates the
effect of any variability in atmospheric d
13
C(d
13
C
air
) in plant
isotopic variation
D ¼
d
13
C
air
d
13
C
plant
1 þ d
13
C
plant
d
13
C
air
d
13
C
plant
ð2Þ
Terrestrial environments
Most paleoclimate inferences from d
13
C in terrestrial settings
are based on plant matter that acquires an isotopic composition
related to the environment in which the plants grew. This signal
may be subsequently passed on to other elements of the Earth
system, to humans/animals from food consumption or to soils
during plant respiration and decomposition of plant matter, for
example. Temperature-dependent equilibrium reactions usually
play a minor role, if any, in the source of the primary environ-
mental signals in these materials.
Terrestrial vegetation is dominated by plants with the C
3
(Calvin-Benson) photosynthetic pathway for which carbon
isotope composition is modeled by the equation of Farquhar
et al. (1982):
d
13
C
plant
¼ d
13
C
air
a ðb aÞ
C
i
C
a
ð3Þ
where d
13
C
air
= d
13
C of the atmospheric CO
2
;C
i
/C
a
= ratio
of leaf intercellular CO
2
concentration to the atmospheric con-
centration; a = fractionation during CO
2
diffusion through sto-
mata (4.4%); and b = enzymatic fractionation by ribulose
CARBON ISOTOPES, STABLE 133