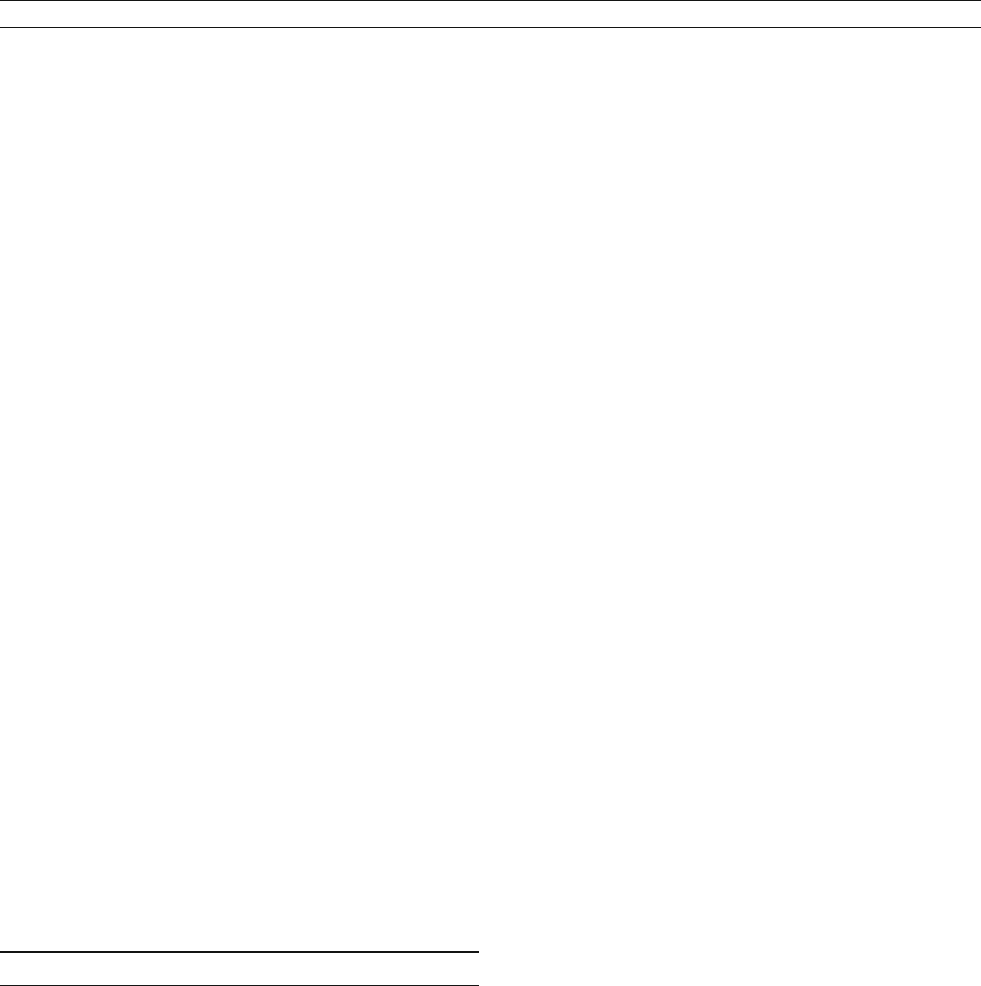
Kasting, J.F., Richardson, S.M., Pollack, J.B., and Toon, O.B., 1986. A
hybrid model of the CO
2
geochemical cycle and its application to large
impact events. Am. J. Sci., 286, 361–389.
Kring, D.A., and Durda, D.D., 2002. Trajectories and distribution of mate-
rial ejected from Chicxulub impact crater: Implications for postimpact
wildfires. J. Geophys. Res., 107, 6-1–6-22.
McKay, C.P., and Thomas, G.E., 1982. Formation of noctilucent clouds by
an extraterrestrial impact. Geol. Soc. Am. Spec. Pap., 190,211–214.
Melosh, H.J., Schneider, N.M., Zahnle, K.J., and Latham, D., 1990. Igni-
tion of global wildfires at the Cretaceous/Tertiary boundary. Nature,
343, 251–254.
Monechi, S., Buccianti, A., and Gardin, S., 2000. Biotic signals from
nannoflora across the iridium anomaly in the upper Eocene of the
Massignano section: Evidence from statistical analysis. Mar. Micropa-
leontol., 39, 219–237.
Pierazzo, E., Kring, D.A., and Melosh, H.J., 1998. Hydrocode simulation
of the Chicxulub impact event and the production of climatically active
gases. J. Geophys. Res., 103, 28,607–28,625.
Pope, K.O., 2002. Impact dust not the cause of the Cretaceous-Tertuiary
mass extinction. Geology, 30,99–102.
Rampino, M.R., 1995. Catastrophe: impact of comets and asteroids.
In Henderson-Sellers, A. (ed.), Future Climates of the World: A Model-
ling Perspective, World Survey of Climatology, vol. 16. Amsterdam:
Elsevier, pp. 95–147.
Rampino, M.R., and Haggerty, B.M., 1994. Extraterrestrial impacts and
mass extinctions of life. In Gehrels, T. (ed.), Hazards Due to
Comets and Asteroids. Tucson: University of Arizona Press,
pp. 827–857.
Rampino, M. R., and Volk, T., 1988. Mass extinctions, atmospheric sulphur
and climatic warming at the K/T boundary. Nature, 332.
Toon, O.B., et al., 1997. Environmental perturbations caused by the impacts
of asteroid and comets. Rev. Geophys., 35,41–78.
Vonhof, H.B., Smit, J., Brinkhuis, H., Montanari, A., and Nederbragt, A.J.,
2000. Global cooling accelerated by early late Eocene impacts. Geol-
ogy, 28, 687–690.
Wolbach, W.S., Gilmour, I., Anders, E., Orth, C.J., and Brooks, R.R., 1988.
Global fire at the Cretaceous-Tertiary boundary.
Nature, 334, 670.
Wolfe, J.A., 1990. Palaeobotanical evidence for a marked temperature
increase following the Cretaceous/Tertiary boundary. Nature, 343,
153–156.
Wolfe, J.A., 1991. Palaeobotanical evidence for a June “impact winter” at
the Cretaceous /Tertiary boundary. Nature, 352, 420–423.
Cross-references
Albedo Feedbacks
Cretaceous/ Tertiary (K /T) Boundary Impact, Climate Effects
Paleoclimate Modeling, Pre-Quaternary
Paleoclimate Modeling, Quaternary
BØLLING-ALLERØD INTERSTADIAL
Introduction
The Bølling-Allerød interstadial is the initial warm phase dur-
ing the Weichselian late glacial that is followed by the cold
Younger Dryas stadial.
The Weichselian late glacial, often referred to as last
glacial-interglacial transition or last termination (ca. 13,000–
10,000
14
CyrBP), was a period of rapid climate change. The
late glacial is classically sub-divided into a series of stadials
named after the occurrence of the characteristic arctic-alpine
plant species Dryas octopetala, separated by interstadials named
after the type localities Bølling and Allerød in Denmark. The
biostratigraphic sub-division has been extensively used in north-
west Europe, usually in a chronostratigraphic sense, and is still
widely used globally.
History
Hartz and Milthers (1901) showed that, based on deposits in a
clay-pit near Allerød (Denmark), a climatic warming had
occurred during the late glacial. Between clay layers containing
mountain avens (Dryas octopetala) and reindeer fossils, an
organic layer containing birch (Betula) and elk fossils was
present, indicating a change from tundra to a more forested
environment followed by a return to tundra. Based on palynol-
ogy, Jessen (1935) was able to define three subsequent pollen
zones: Early Dryas (I), Allerød (II), and Late Dryas (III). This
subdivision was further refined by Iversen (1942), who added
another interstadial phase that supposedly occurred before the
Allerød and was first described in Bølling Sø (Denmark).
The palynological sub-division of the Weichselian late glacial
established by Jessen ( 1935) and Iversen (1942) was introduced
into The Netherlands by van der Hammen, who recognized the
Bølling and Allerød oscillations, separated by the Dryas stadials
in a site named Hijkermeer. The interstadial deposits were char-
acterized by a higher content of organics in comparison with
the stadial deposits. Van der Hammen (1951) went on to demon-
strate a similar vegetation development at different locations
in The Netherlands. Since then, hundreds of pollen diagrams
from late glacial deposits have been constructed using van der
Hammen’s scheme (Hoek, 1997).
Bio- and chronostratigraphy
Unfortunately, the boundaries of similar biostratigraphical zones
defined in different regions are in most cases diachronous due to
differences in vegetation response to changes in climate. This
makes the use of the biostratigraphic zones defined in Scandinavia
problematic outside this region. Furthermore, there are problems
that can be regarded as inconsistencies in biostratigraphic defini-
tion. In northwestern Germany especially, an additional intersta-
dial called Meiendorf is recognized, which correlates with the
Bølling interstadial, while the term Bølling is used for the first
partof the Allerød interstadial (Litt and Stebich, 1999). The discre-
pancy between the definition of Bølling and Allerød is mainly the
result of a difference of opinion about the correlation with
the section at Bølling Sø, first studied by Iversen (1942). In
Britain, it is often difficult to recognize stadial conditions separ-
ating the individual interstadial phases (Lowe and Gray, 1980).
Terms such as late glacial interstadial or Windermere interstadial
have been used for the Bølling-Allerød interstadial complex.
These examples all point towards the problems of transferring
the biostratigraphic zonation scheme from one region to another.
A detailed discussion regarding this issue is presented by de
Klerk (2004).
With the introduction of the radiocarbon dating method, bios-
tratigraphic correlation became less important and pollen diagrams
were considered more frequently in a chronological context.
Moreover, the terminology that was originally developed for bios-
tratigraphical zones (Bølling, Allerød, etc.) has been used in a
chronostratigraphic sense (Mangerud et al., 1974). Since then,
the terms Bølling and Allerød have been connected worldwide
to the time period between 13,000 and 11,000 yr
BP, as defined
by Mangerud et al. (1974). This chronological definition was,
however, initially based on the radiocarbon-dated biostratigraphic
zone boundaries from southern Scandinavia. Above this, the
Mangerud et al. (1974) scheme has been constructed using bulk
radiocarbon dates and was constructed when reservoir ages and
radiocarbon-plateaux were not yet known (Wohlfarth, 1996).
If the chronostratigraphic scheme is compared to the
14
C-dated
100 BØLLING-ALLERØD INTERSTADIAL