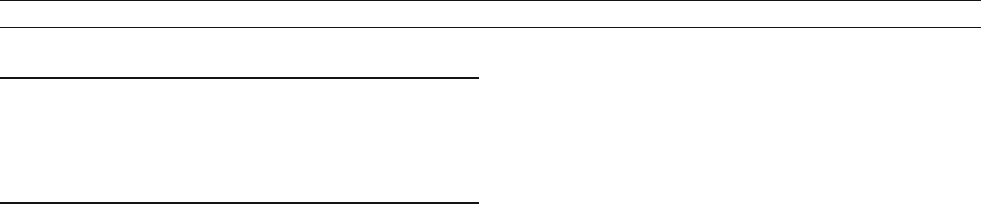
significant variations in wind speed and strength during stadials
and interstadials (Biscaye et al., 1997).
Zeolites
Zeolites (Table M3) are a group of hydrated aluminosilicate
minerals with an open framework structure, into which various
large ions and water molecules can fit and which possess a cer-
tain degree of mobility. They are widespread in cavities in
basaltic lavas, as authigenic or diagenetic minerals in marine
sediments, and in low-grade metamorphic rocks. Phillipsite is
an authigenic zeolite that forms on the seafloor or at shallow
depths (Kastner, 1999) Clinoptilolite forms in silica-rich marine
sediments, derived from opal-A. Zeolites also form from the
alteration of volcanic glass, tuff, or lavas in high pH, alkali-
rich, semi-arid to arid environments. Analcime is common in
sodium-rich alkaline desert soils (Watson, 1992). Zeolites from
saline, alkaline lakes include analcime, chabazite, clinoptilolite,
erionite, mordenite, and phillipsite. These zeolites are often
associated with evaporites (Parrish, 1998). Zeolites in lacus-
trine deposits have been used as markers for dry, alkaline
environments, as for example at Lake Naivasha, Kenya, where
low lake levels are represented by the presence of chabazite
and phillipsite, with even greater aridity indicated by clinoptilo-
lite and analcime (Trauth et al., 2001). The East African lake
level fluctuations have been linked to precessional cycles in
springtime insolation and monsoonal precipitation.
Summary
Minerals that form at or near the earth’s surface in contact with
the atmosphere and hydrosphere can provide important infor-
mation about former climates. They have been used to recon-
struct past climates and changes over time, deduce changes in
atmospheric composition, trace eolian and precipitation pat-
terns, and serve as “hosts” for climate-sensitive stable isotopes
and trace elements.
The most useful mineral indicators or proxies are those that
are generated under relatively narrow climatic ranges or within
restricted environmental settings. Examples include evaporites,
low temperature minerals (e.g., ikaite and hydrohalite), residual
soils (e.g., iron and aluminum oxyhydroxides, kaolinite), and
certain authigenic minerals (that form in situ, e.g., glauconite,
bertherine, phillipsite, barite). Minerals that are sensitive to oxi-
dation (e.g., pyrite and uraninite) reflect past atmospheric oxy-
gen levels. Distinctive detrital minerals in eolian deposits act as
markers of wind direction and intensity. Similarly, minerals in
deltaic sediments point not only to variations in the relative
contributions from source tributaries, but can also indicate pre-
cipitation variability in source areas. Trace elements and stable
isotope ratios contained within minerals such as calcite (both
biogenic and non-biogenic) have provided a wealth of paleocli-
matological information.
Vivien Gornitz
Bibliography
Benison, K.C., and Goldstein, R.H., 1999. Permian paleoclimate data from
fluid inclusions in halite. Chem. Geol., 154,113–132.
Biscaye, P.E., Grousset, F.E., Revel, M., Van der Gaast, Zielinski, G.A.,
Vaars, A., and Kukla, G., 1997. Asian provenance of glacial dust (stage 2)
in the Greenland Ice Sheet Project 2 ice core, Summit, Greenland.
J. Geophys. Res., 10226,765–26, 781.
Bond, G., Showers, W., Cheseby, M., Lotti, R., Almasi, P., deMenocal, P.,
Priore, P., Cullen, H., Hajdas, I., and Bonani, G., 1997. Massive millen-
nial-scale cycle in North Atlantic Holocene and Glacial climates.
Science, 278, 1257–1266.
Broecker, W.S., and Liu, T., 2001. Rock varnish: recorder of desert
wetness? GSA Today, 11(8), 10.
Chamley, H., 1989. Clay Sedimentology. Berlin: Springer, 623p.
De Lurio, J.l., and Frakes, L.A., 1999. Glendonites as a paleoenvironmental
tool: implications for early Cretaceous high latitude climates in
Australia. Geochim. et Cosmochim. Acta, 63, 1039–1048.
Derry, L.A., and Murray, R.W., 2004. Continental margins and the
sulfur cycle. Science, 303, 1981–1982.
England, G.L., Rasmussen, B., Krapez, B., and Groves, D.I., 2002.
Palaeoenvironmental significance of rounded pyrite in siliciclastic
sequences of the Late Archean Witwatersrand Basin: Oxygen-
deficient atmosphere or hydrothermal alteration? Sedimentology, 49
(6), 1133–1156.
Evans, L.J., 1992. Alteration products at the Earth’s surface–the clay
minerals. In Martini, I.P., and Chesworth, W. (eds.), Weathering, Soils,
and Paleosols, Amsterdam: Elsevier, Chap. 5, pp. 107–125.
Fleet, M.E., 1998. Detrital pyrite in Witwatersrand gold reefs: X-ray
diffraction evidence and implications for atmospheric evolution. Terra
Nova, 10(6), 302–306.
Foucault, A., and Stanley, D.J., 1989. Late Quaternary palaeoclimatic
oscillations in East Africa recorded by heavy minerals in the Nile delta.
Nature, 339,44–46.
Genty, D., Blamart, D., Ouahdi, R., Gilmour, M., Baker, A., Jouzel, J., and
Van-Exter, S., 2003. Precise dating of Dansgaard-Oeschger climate
oscillations in western Europe from stalagmite data. Nature, 421,
833–837.
Gornitz, V.M., and Schreiber, B.C., 1981. Displacive halite hoppers
from the Dead Sea: implications for ancient evaporite deposits. J. Sed.
Petrol., 51, 787–794.
Hessler, A.M., Lowe, D.R., Jones, R.L., and Bird, D.K., 2004. A
lower limit for atmospheric carbon dioxide levels 3.2 billion years
ago. Nature, 428, 736–738.
Kastner, M., 1999. Oceanic minerals; their origin, nature of their environ-
ment, and significance. Proc. Natl. Acad. Sci., 96, 3380–3387.
Kennedy, M., Droser, M, Mayer, L.M., Pevear, D., and Mrofka, D., 2006.
Late Precambrian oxygenation; inception of the clay mineral factory.
Science, 311, 1446–1449.
Kirk, J., Ruiz, J., Chesley, J., and Titley, S., 2003. The origin of gold in
South Africa. Am. Scientist, 91, 536–541.
Li, Y.H., and Schoonmaker, J.E., 2003. Chemical composition and
mineralogy of marine sediments. In: Mackenzie F.T. (ed.), Sediments,
Diagenesis, and Sedimentary Rocks (vol. 7), Treatise of Geochemistry,
Holland, H.D., and Turekian, K.K. (eds.). Amsterdam: Elsevier,
pp. 1–35.
Lowenstein, T.K., Timofeeff, M.N., Brennan, S.T., Hardie, L.A., and
Demicco, R.V., 2001. Oscillations in Phanerozoic seawater chemistry:
Evidence from fluid inclusions. Science, 294, 1086–1088.
Ludvigson, G.A., Gonzalez, L.A., Metzger, R.A., Witzke, B.J., Brenner, R.L.,
Murillo, A.P., and White, T.S., 1998. Meteoric sphaerosiderite lines and
their use for paleohydrology and paleoclimatology. Geology, 26,
1039–1042.
Ludvigson, G.A., Ufnar, D.F., Gonzalez, L.A., White, T.S., Phillips, P.L.,
Witzke, B.J., and Brenner, R.L., 2000. When good sphaeorosiderites
go bad: Caveats in the application of a paleoclimate proxy. Geol. Soc.
Am. Abstr. Prog., 32(7), pp. A–524.
Maher, B.A., 1998. Magnetic properties of modern soils and Quaternary
loessic paleosols: paleoclimatic implications. Palaeogeog. Palaeoclim.
Palaeoecol., 137,25– 54.
Mallinson, D.J., Flower, B., Hine, A., Brooks, G., and Garza, R.M., 2003.
Paleoclimate implications of high latitude precession-scale mineralogic
fluctuations during early Oligocene Antarctic glaciation: The Great
Australian Bight record. Glob. Planet. Change, 39, 257–269.
Table M3 Common zeolite minerals
Analcime NaAlSi
2
O
6
·H
2
O
Chabazite Ca
2
(Al
4
Si
8
O
24
)·12H
2
O
Clinoptilolite (Na,K,Ca
0.5
)
6
(Al
6
Si
30
O
72
)·24H
2
O
Erionite (Na,K
2
,Mg,Ca
1.5
)(Al
8
Si
28
O
72
)·28H
2
O
Mordenite Na
3
KCa
2
(Al
8
Si
40
O
96
)·28H
2
O
Phillipsite (K,Ca
0.5
,Na)
4
(Al
6
Si
10
O
32
)·12H
2
O
582 MINERAL INDICATORS OF PAST CLIMATES