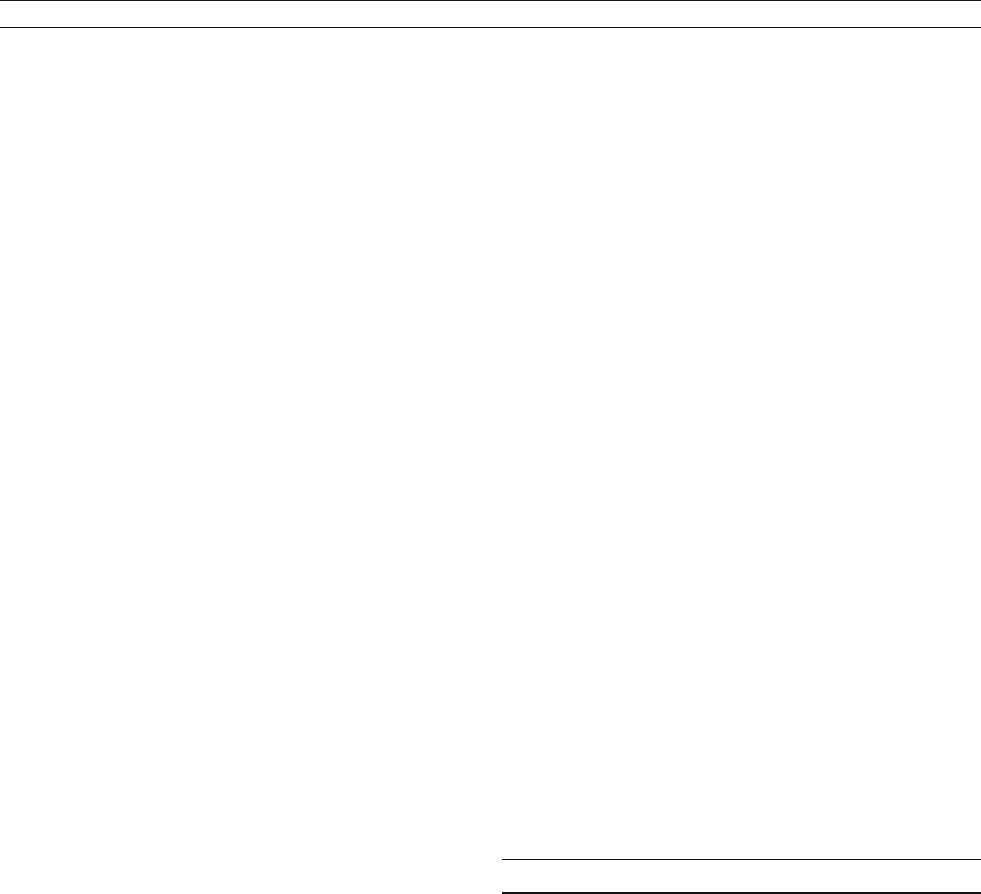
all mass extinctions have a single underlying cause is unclear.
Temperature change appears to be one common theme to
the five major mass extinctions of the Phanerozoic, but the
driving mechanism(s) can differ between extinctions. The Late
Ordovician extinction, for example, is clearly related to a gra-
dual decline in global temperatures, while the Cretaceous-
Tertiary boundary extinctions are related (at least in part) to
catastrophic temperature decline resulting from bolide impact.
Each mass extinction event probably has its own unique set
of environmental changes.
Further understanding of mass extinction will require an
interdisciplinary effort involving geologists, paleontologists,
geochemists, and geophysicists.
Garland R. Upchurch, Jr.
Bibliography
Alvarez, L.W., Alvarez, W., Asaro, F., and Michel, H., 1980. Extraterres-
trial cause for the Cretaceous-Tertiary extinction. Experimental results
and theoretical interpretation. Science, 208, 1095–1108.
Beerling, D.J. et al., 2001. Evidence for the recovery of terrestrial eco-
systems ahead of marine primary production following a biotic crisis
at the Cretaceous-Tertiary boundary. J. Geol. Soc. Lond., 158, 737–740.
Beerling, D.J., Lomax, B.H., Royer, D.L., Upchurch, G.R., and
Kump, L.R., 2002. An atmospheric pCO
2
reconstruction across the
Cretaceous-Tertiary boundary from leaf megafossils. Proc. Natl. Acad.
Sci. USA, 99, 7836–7840.
Berner, R.A., 2002. Examination of hypotheses for the Permo-Triassic
boundary extinction by carbon cycle modeling. Proc. Natl. Acad. Sci.
USA, 99, 4172–4177.
Berner, R.A., Beerling, D.J., Dudley, R., Robinson, J.M., and Wildman, W.
A., 2003. Phanerozoic atmospheric oxygen. Annu. Rev. Earth Planet.
Sci., 31, 105–134.
Clemens, W.A., 1982. Patterns of extinction and survival of the terrestrial
biota during the Cretaceous /Tertiary transition. Geol. Soc. Am. Spec.
Pap., 190, 407–414.
Covey, C., Thompson, S.L., Weissman, P.R., and MacCracken, M.C., 1994.
Global climatic effects of atmospheric dust from an asteroid or comet
impact on Earth. Glob. planet. Change, 9, 263–273.
Crowley, T.J., 2000. Carbon dioxide and Phanerozoic climate. In
Huber, B.T., MacLeod, K.G., and Wing, S.L. (eds.), Warm Climates
in Earth History. Cambridge, UK: Cambridge University Press,
pp. 425–444.
Emanuel, K.A., 2003. Tropical cyclones. Annu. Rev. Earth Planet. Sci., 31,
75–104.
Erwin, D.H., 1993. The Great Paleozoic Crisis: Life and Death in the
Permian. New York, NY: Columbia University Press, 327pp.
Gibbs, M.T., Bice, K.L., Barron, E.J., and Kump, L.R., 2000. Glaciation
in the early Paleozoic ‘greenhouse’: the roles of paleogeography and
atmospheric CO
2
. In Huber, B.T., MacLeod, K.G., and Wing, S.L.
(eds.), Warm Climates in Earth History. Cambridge, UK: Cambridge
University Press, pp. 386–422.
Hallam, A., and Wignall, P.B., 1997. Mass Extinctions and Their
Aftermath. Oxford, UK: Oxford University Press, 320pp.
Hesselbo, S.P., Robinson, S.A., Surlyk, F., and Piasecki, S., 2002.
Terrestrial and marine extinction at the Triassic-Jurassic boundary
synchronized with major carbon-cycle perturbation: A link to the initia-
tion of massive volcanism? Geology, 30, 251–254.
Kaiho, K., Kajiwara, Y., Tazaki, K., Ueshima, M., Takeda, N.,
Kawahata, H., Arinobu, T., Ishiwatari, R., Hirai, A., and Lamolda, M.A.,
1999. Oceanic primary productivity and dissolved oxygen levels at the
Cretaceous/ Tertiary boundary; their decrease, subsequent warming, and
recovery. Paleoceanography, 14,511–524.
Keller, G., Stinnesbeck, W., Adatte, T., and Stuben, D., 2003. Multiple
impacts across the Cretaceous-Tertiary boundary. Earth Sci. Rev., 62,
327–363.
Kump, L.R., and Arthur, M.A., 1999. Interpreting carbon-isotope excur-
sions: carbonates and organic matter. Chem. Geol., 161, 181–198.
Maruoka, T., and Koeberl, C., 2003. Acid-neutralizing scenario after the
Cretaceous-Tertiary boundary impact event. Geology, 31, 489–492.
McGhee, G.R., 1996. The Late Devonian Mass Extinction: The Frasnian/
Famennian Crisis. New York, NY: Columbia University Press, 303pp.
Olsen, P.E., Kent, K.V., Sues, H.-D., Koeberl, C., Huber, H., Montanari, A.,
Rainforth, E.C., Fowell, S.J., Szajna, M.J., and Hartline, B.W., 2002.
Ascent of dinosaurs linked to an iridium anomaly at the Triassic-
Jurassic boundary. Science, 296, 1305–1307.
Rampino, M.R., Self, S., and Stothers, R.B., 1988. Volcanic winters. Annu.
Rev. Earth Planet. Sci., 16,73–99.
Royer, D.L., Berner, R.A., and Beerling, D.J, 2001. Phanerozic CO
2
change: Evaluating geochemical and paleobiological approaches. Earth
Sci. Rev., 54, 349–392.
Ryskin, G., 2003. Methane-driven oceanic eruptions and mass extinctions.
Geology, 31, 741–744.
Sheehan, P.M., 2001. The late Ordovician mass extinction. Annu. Rev.
Earth Planet. Sci., 29, 331–364.
Sheehan, P.M., and Hansen, T.A., 1986. Detritus feeding as a buffer to
extinction at the end of the Cretaceous. Geology, 14, 868–870.
Stanley, S.M., 1984. Temperature and biotic crises in the marine realm.
Geology, 12, 205–208.
Toon, O.B., Zahnle, K., Morrison, D., Turco, R.P., and Covey, C., 1997.
Environmental perturbations caused by the impacts of asteroids and
comets. Rev. Geophys., 35,41–78.
Upchurch, G.R., 1989. Terrestrial environmental changes and extinction
patterns at the Cretaceous-Tertiary boundary, North America. In
Donovan, S.K. (ed.), Mass Extinctions: Processes and Evidence.
London, UK: Belhaven Press, pp. 195–216.
Wignall, P.B., 2001. Large igneous provinces and mass extinctions. Earth
Sci. Rev., 53,1–33.
Wolfe, J.A., 1990. Palaeobotanical evidence for a marked temperature
increase following the Cretaceous/Tertiary boundary. Nature, 343,
153–156.
Cross-references
Bolide Impacts and Climate
Carbon Cycle
Carbon Isotope Variations Over Geologic Time
Cretaceous/ Tertiary (K-T) Boundary Impact, Climate Effects
Flood Basalts: Climatic Implications
Late Paleozoic Paleoclimates (Carboniferous-Permian)
Methane Hydrates, Carbon Cycling, and Environmental Change
Paleobotany
Volcanic Eruptions and Climate Change
MAUNDER MINIMUM
The Maunder Minimum refers to the period from about 1645 to
1715 during which very few sunspots were observed. Follow-
ing Galileo’s refinement of the telescope around 1610, observa-
tions of sunspots showed that they appeared to be a fairly
regular phenomenon. One of the main features was that their
abundances waxed and waned approximately every 11 years,
which became known as the solar cycle or sunspot cycle. Dur-
ing the Maunder Minimum, however, relatively very few sun-
spots were seen for roughly seventy years, and the 11-year
cycle was dramatically reduced (Eddy, J.A., 1976). After 1715,
the cycle built up again, and such a dramatic decrease in sun-
spots has not been seen since. When the sun is more active there
are a greater number of sunspots. Though these are dark patches
that emit less radiation than normal, their abundance is correlated
with overall greater solar output since bright spots (faculae) are
more abundant at the same times. The Maunder Minimum, with
an extremely quiescent sun, is therefore thought to have been a
low point for solar output. This may have contributed to colder
climate conditions over many regions of the Earth during this
550 MAUNDER MINIMUM