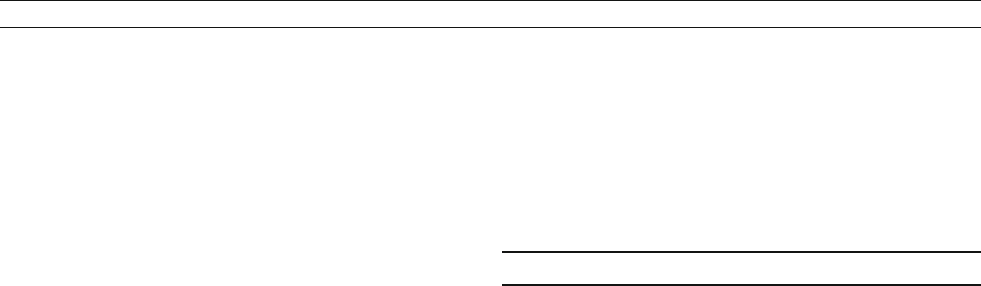
Bond, G.C., Showers, W., Cheseby, M., Lotti, R., Almasi, P., deMenocal, P.,
Priore, P., Cullen, H., Hajdas, I., and Bonani, G., 1997. A pervasive mil-
lennial-scale cycle in North Atlantic Holocene and Glacial climates.
Science, 278, 1257–1266.
Bond, G.C., Showers, W., Elliot, M., Evans, M., Lotti, R., Hajdas, I.,
Bonani, G., and Johnson, S., 1999. The North Atlantic’s1–2 Kyr cli-
mate rhythm: Relation to Heinrich events, Dansgaard/ Oeschger cycles
and the Little Ice Age. In Clark, P.U., Webb, R.S., and Keigwin, L.D.
(eds.), Mechanisms of global climate change at millennial time scales.
Washington, DC: AGU, Geophysical Monograph Series 112, pp.
35–58.
Bond, G.C., Kromer, B., Beer, J., Muscheler, R., Evans, M., Showers, W.,
Hoffmann, S., Lotti-Bond., R., Hajdas, I., and Bonani, G., 2001. Persis-
tent solar influence on North Atlantic climate during the Holocene.
Science, 294, 2130–2136.
Braun, H., Christl, M., Rahmstorf, S., Ganopolski, A., Mangini, A.,
Kubatzki, C., Roth, K., and Kromer, B., 2005. Possible solar origin
of the 1,470-year glacial climate cycle demonstrated in a coupled
model. Nature, 438, 208–211.
Broecker, W.S., 1994. Massive iceberg discharges as triggers for global
climate change. Nature, 372, 421–424.
Broecker, W.S., Bond, G., Klas, M., Bonani, G., and Wolfl, W., 1990.
A salt oscillator in the glacial North Atlantic? 1. The concept. Paleocea-
nography, 5, 469–477.
Broecker, W.S., Bond, G.C., Klas, M., Clark, E., and McManus, J.F., 1992.
Origin of the northern Atlantic’s Heinrich events. Clim. Dynam., 6,
265–273.
Ganopolski, A., and Rahmstorf, S., 2001. Rapid changes of glacial climate
simulated in a coupled climate model. Nature, 409, 153–158.
Grachev, A., and Severinghaus, J., 2005. A revised +10 4
C magnitude
of the abrupt change in Greenland temperature at the Younger Dryas
termination using published GISP2 gas isotope data and air thermal
diffusion constants. Quaternary Sci. Rev., 24, 513–519.
Greve, R., and MacAyeal, D.R., 1996. Dynamic/thermodynamic simula-
tions of Laurentide ice-sheet instability. Ann. Glaciol., 23, 328–335.
Heinrich, H., 1988. Origin and consequences of cyclic ice rafting in the
northeast Atlantic Ocean during the past 130,000 years. Quaternary
Res., 29, 142–152.
Hemming, S.R., 2004. Heirich events: Massive late Pleistocene detritus
layers of the North Atlantic and their global climate imprint. Rev.
Geophys., 42,1–43.
Hulbe, C.L., MacAyeal, D.R., Denton, G.H., Kleman, J., Lowell, T.V.,
2004. Catastrophic ice shelf breakup as the source of Heinrich
event icebergs. Paleoceanography, 19(1). doi: PA1004 10.1029/
2003PA000890.
Landais, A., Barnola, J.M., Masson-Delmotte, V., Jouzel, J., Chappellaz, J.,
Caillon, N., Huber, C., Leuenberger, M., and Johnsen, S.J., 2004. A
continuous record of temperature evolution over a sequence of
Dansgaard-Oeschger events during marine stage 4 (76–62 ky
BP).
Geophys. Res. Lett., 31,1–4, L22211. doi: 10.1029 /2004GL021193.
MacAyeal, D.R., 1993. Binge /purge oscillations of the Laurentide ice
sheet as a cause of the North Atlantic’s Heinrich events. Paleoceano-
graphy, 8(6), 775–784.
Mayewski, P.A., Meeker, L.D., Twickler, M.S., Whitlow, S., Yang, Q.,
Lyons, W.B., and Prentice, M., 1997. Major features and forcing of
high-latitude Northern Hemisphere atmospheric circulation using
a 110,000 year-long glaciochemical series. J. Geophys. Res., 102,
26345–26366.
North Greenland Ice Core Project Members, 2004. High-resolution
record of northern hemisphere climate extending into the last intergla-
cial period. Nature, 431, 147–157.
Rahmstorf, S., 2003. Timing of abrupt climate change: A precise clock.
Geophys. Res. Lett., 30(10), 1510. doi: 10,1029/2003GL.017115.
Schulz, M., and Paul, A., 2002. Holocene climate variability on centennial-
to-millennial time scales 1. Climate records from the North-Atlantic
realm. In Wefer, G., Berger, W.H., Behre, K.-E. and Jansen, E. (eds.),
Climate Development and History of the North Atlantic Realm. Berlin:
Springer, pp. 41–54.
Stott, L., Poulsen, C., Lund, S., and Thunell, R., 2002. Super ENSO
and global climate oscillations at millennial time scales. Science, 297,
222–226.
Voelker, A.H.L., 2002. Global distribution of centennial-scale records
for marine isotope stage (MIS) 3: A database. Quaternary Sci. Rev.,
21(10), 1185–1212.
Cross-references
Binge-Purge Cycles of Ice Sheet Dynamics
Dansgaard-Oeschger Cycles
Heinrich Events
Ice-Rafted Debris (IRD)
Quaternary Climate Transitions and Cycles
Sun-Climate Connections
Younger Dryas
MINERAL INDICATORS OF PAST CLIMATES
Introduction
A mineral is a naturally occurring crystalline solid created by
geological or biogenic processes (e.g., calcite/aragonite in mol-
lusk shells or coral). Minerals that form at or near the Earth’ssur-
face are products of chemical weathering, evaporation, authigenic
crystallization, and bio-mineralization. They reflect ambient con-
ditions at the Earth-atmosphere interface. Therefore they can fur-
nish important clues about former climates. Minerals are utilized
as paleoclimate indicators or proxies in several different ways:
1. To infer past climates and changes over time.
2. To deduce changes in atmospheric composition.
3. As mineralogical “markers” in provenance studies.
4. As “hosts” for climate-sensitive stable isotopes and trace
elements.
The most direct mineral indicators or proxies are those that
are generated under relatively narrow climatic ranges or within
restricted environmental settings. Examples include chemical
precipitates such as evaporites, low temperature minerals such
as ikaite and hydrohalite, residual soils (iron and aluminum
oxyhydroxides, kaolinite), and authigenic minerals (forming
in situ, e.g., glauconite, bertherine, phillipsite, barite). Evapor-
ite minerals (e.g., gypsum, anhydrite, halite, sylvite) are gener-
ally indicative of arid to hyperarid climates. They form in
restricted marine basins, narrow rift valleys, coastal lagoons,
sabkhas (salt flats), and internally-drained continental interiors,
where rates of evaporation exceed rates of water supplied by
rivers or rainfall (McLane, 1995; Parrish, 1998; Evaporites).
The distribution of modern evaporites closely corresponds
to the distribution of deserts. In addition, diagnostic of aridity
are chemically-resistant minerals like quartz that are concen-
trated into eolian sand deposits (and ultimately sandstones) by
wind transport and deposition. Sedimentary rocks of biogenic
origin (e.g., chalks, cherts, phosphorites) provide information
on paleotemperatures, productivity, and ocean circulation.
Clay minerals derived from chemical weathering of rocks are
relevant climate indicators, since they denote prevailing condi-
tions at the time of formation. Kaolinite, for example, although
widespread in many soils, is characteristic of tropical weather-
ing, whereas chlorite is usually more abundant in colder
regimes, where physical weathering predominates. Changes in
composition of marine or lacustrine clays often parallel climate
fluctuations (e.g., Mallinson et al., 2003; Robert and Kennett,
1997; Yuretich et al., 1999, see below).
Minerals that are sensitive to oxidation may provide infor-
mation about past atmospheres. Pyrite and uraninite oxidize
and alter rapidly under current atmospheric conditions. Their
preservation in detrital grains from 3 billion year old sediments
implies much lower atmospheric oxygen than at present (Fleet,
MINERAL INDICATORS OF PAST CLIMATES 573