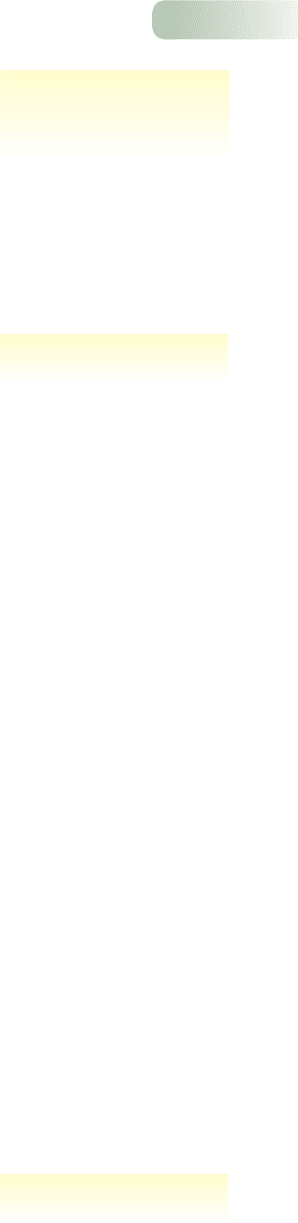
The clearing and burning of tropical forest to make way for agriculture or
timber production and the decay of the residues make a further contribution to
the increase in atmospheric carbon dioxide (Table 13.1). A considerable amount
of this is recaptured in photosynthesis by the replacement vegetation (Kicklighter
et al., 1999), but this is least when forest is converted to grassland, which has
a much lower biomass. In total about 1.0 × 10
9
metric tons per year has been
released through changes in tropical land use (Detwiler and Hall, 1988). This
calculation was made for 1980, and the figure for tropical forest clearance must
now be significantly greater as a result of the uncontrollable spread of forest
fires in Indonesia and in South America following the droughts associated with
the El Niño phenomenon of 1997/98.
The Earth’s atmosphere behaves like a greenhouse. Solar radiation warms
up the Earth’s surface, which reradiates energy outward, principally as infrared
radiation. Carbon dioxide – together with other gases whose concentrations
have increased as a result of human activity (nitrous oxide, methane, ozone,
chlorofluorocarbons) – absorbs infrared radiation. Like the glass of a greenhouse,
these gases (and water vapor) prevent some of the radiation from escaping and keep
the temperature high. The air temperature at the land surface is now 0.6 ± 0.2°C
warmer than in pre-industrial times. Given further predicted rises in greenhouse
gases, temperatures will continue to rise by a global average of between 2.0°C and
5.5°C by 2100 (IPCC, 2001; Millennium Ecosystem Assessment, 2005), but to
different extents in different places. Such changes will lead to a melting of glaciers
and icecaps, a consequent rise in sea level, and large changes to global patterns of
precipitation, winds, ocean currents and the timing and scale of storm events.
In response to these changes, we can expect latitudinal and altitudinal shifts
in species distributions and widespread extinctions as floras and faunas fail to
track and keep up with the rate of change in global temperatures (Hughes, 2000).
In addition, the global threats imposed by harmful invasive species will change.
Take, for example, the Argentine ant (Linepithema humile), a native of South
America. This is now established on every continent except Antarctica. It can
achieve extremely high densities and has adverse consequences for biodiversity
(eliminating native invertebrates) and for domestic life, swarming over human
foodstuffs and even sleeping babies. A distributional model was developed for
the ant, based on occurrences in its native and invaded ranges, and related both
to climatic data (e.g. maximum, minimum and mean temperatures, precipitation,
number of frost days, number of wet days) and topographic data (e.g. elevation,
slope, aspect). The model provided a good fit with current distribution based
on current climate. Next, predicted climate change was used to model the ant’s
future distribution. Figure 13.7 indicates in red those areas predicted to improve
for the ant by 2050 (increased likelihood of ant occurrence) and in blue those
areas expected to worsen. The species will retract its range in tropical areas but
expand into higher latitudes. Ironically, the Argentine ant looks set to do less
well in its native South America than in North America and Europe.
Efforts to eradicate Argentine ants have mostly been unsuccessful. The
management response is therefore to increase biosecurity precautions in regions
expected to become progressively more invadable in future.
Of the pollutants that humans release into the atmosphere, most are returned
to Earth, about half as gases or particles and half dissolved or suspended in rain,
snow and fog. They may be carried in the wind for hundreds of kilometers across
Chapter 13 Habitat degradation
437
atmospheric pollution due to
the burning of fossil fuels
and deforestation
the greenhouse effect
acid rain
9781405156585_4_013.qxd 11/5/07 15:03 Page 437