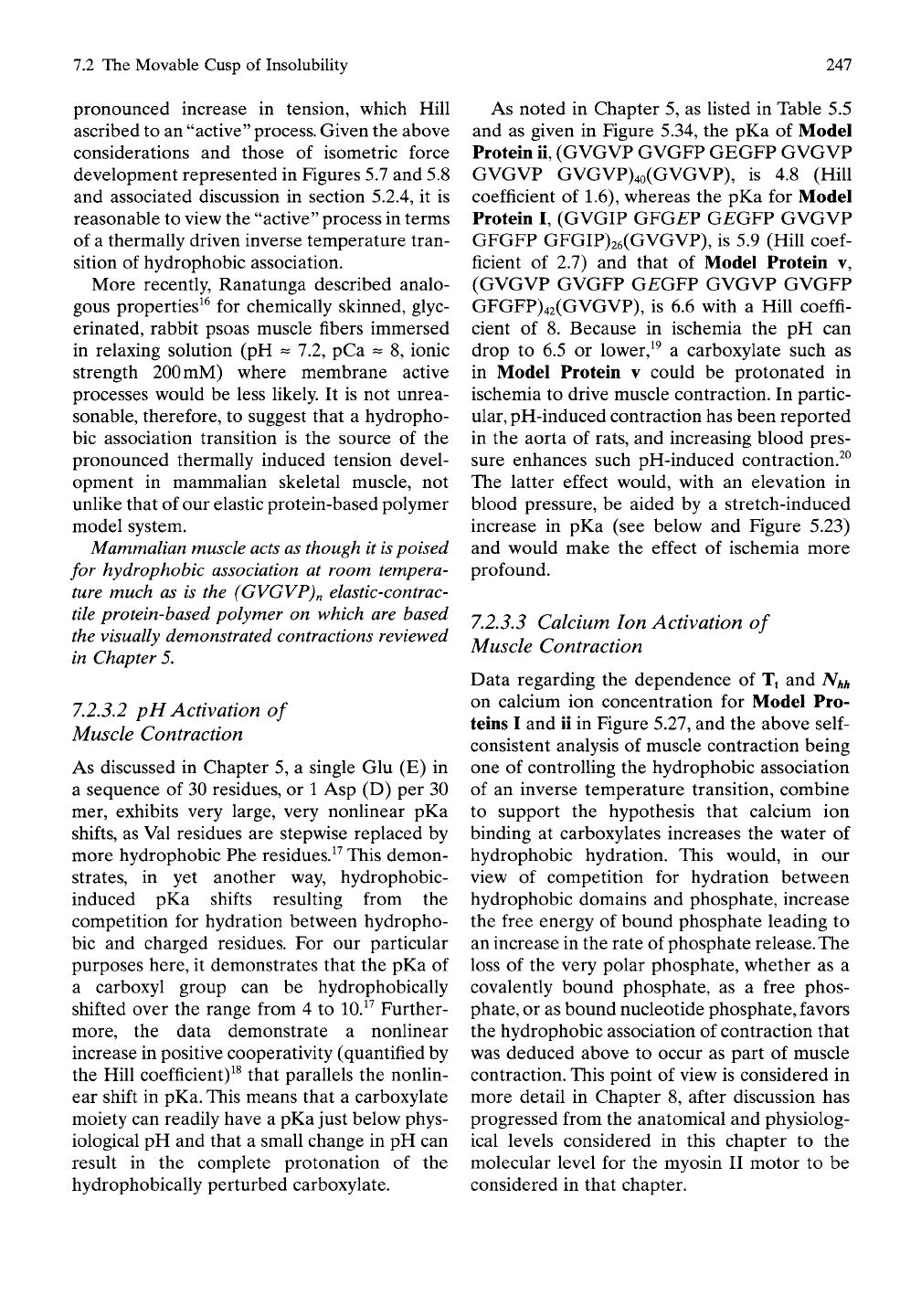
7.2 The Movable Cusp of Insolubility
247
pronounced increase in tension, which Hill
ascribed to an "active" process. Given the above
considerations and those of isometric force
development represented in Figures 5.7 and 5.8
and associated discussion in section 5.2.4, it is
reasonable to view the "active" process in terms
of a thermally driven inverse temperature tran-
sition of hydrophobic association.
More recently, Ranatunga described analo-
gous properties'^ for chemically skinned, glyc-
erinated, rabbit psoas muscle fibers immersed
in relaxing solution (pH ~ 7.2, pCa ~ 8, ionic
strength 200 mM) where membrane active
processes would be less likely. It is not unrea-
sonable, therefore, to suggest that a hydropho-
bic association transition is the source of the
pronounced thermally induced tension devel-
opment in mammahan skeletal muscle, not
unlike that of our elastic protein-based polymer
model system.
Mammalian muscle acts as though it
is
poised
for hydrophobic association at room tempera-
ture much as is the (GVGVP)^ elastic-contrac-
tile protein-based polymer on which are based
the visually demonstrated contractions reviewed
in Chapter 5.
7.2.3,2
pH Activation of
Muscle Contraction
As discussed in Chapter 5, a single Glu (E) in
a sequence of 30 residues, or 1 Asp (D) per 30
mer, exhibits very large, very nonlinear pKa
shifts,
as Val residues are stepwise replaced by
more hydrophobic Phe residues.'^ This demon-
strates, in yet another way, hydrophobic-
induced pKa shifts resulting from the
competition for hydration between hydropho-
bic and charged residues. For our particular
purposes here, it demonstrates that the pKa of
a carboxyl group can be hydrophobically
shifted over the range from 4 to 10.'^ Further-
more, the data demonstrate a nonlinear
increase in positive cooperativity (quantified by
the Hill coefficient)'^ that parallels the nonlin-
ear shift in pKa. This means that a carboxylate
moiety can readily have a pKa just below phys-
iological pH and that a small change in pH can
result in the complete protonation of the
hydrophobically perturbed carboxylate.
As noted in Chapter 5, as listed in Table 5.5
and as given in Figure 5.34, the pKa of Model
Protein ii, (GVGVP GVGFP GEGFP GVGVP
GVGVP GVGVP)4o(GVGVP), is 4.8 (Hill
coefficient of 1.6), whereas the pKa for Model
Protein I, (GVGIP GFG£P G^GFP GVGVP
GFGFP GFGIP)26(GVGVP), is 5.9 (Hill
coef-
ficient of 2.7) and that of Model Protein v,
(GVGVP GVGFP GEGFP GVGVP GVGFP
GFGFP)42(GVGVP), is 6.6 with a Hill coeffi-
cient of 8. Because in ischemia the pH can
drop to 6.5 or lower,^^ a carboxylate such as
in Model Protein v could be protonated in
ischemia to drive muscle contraction. In partic-
ular, pH-induced contraction has been reported
in the aorta of rats, and increasing blood pres-
sure enhances such pH-induced contraction.^^
The latter effect would, with an elevation in
blood pressure, be aided by a stretch-induced
increase in pKa (see below and Figure 5.23)
and would make the effect of ischemia more
profound.
7.2.3.3
Calcium Ion Activation of
Muscle Contraction
Data regarding the dependence of Tt and
NHH
on calcium ion concentration for Model Pro-
teins I and ii in Figure 5.27, and the above
self-
consistent analysis of muscle contraction being
one of controlling the hydrophobic association
of an inverse temperature transition, combine
to support the hypothesis that calcium ion
binding at carboxylates increases the water of
hydrophobic hydration. This would, in our
view of competition for hydration between
hydrophobic domains and phosphate, increase
the free energy of bound phosphate leading to
an increase in the rate of phosphate
release.
The
loss of the very polar phosphate, whether as a
covalently bound phosphate, as a free phos-
phate, or as bound nucleotide phosphate, favors
the hydrophobic association of contraction that
was deduced above to occur as part of muscle
contraction. This point of view is considered in
more detail in Chapter 8, after discussion has
progressed from the anatomical and physiolog-
ical levels considered in this chapter to the
molecular level for the myosin II motor to be
considered in that chapter.