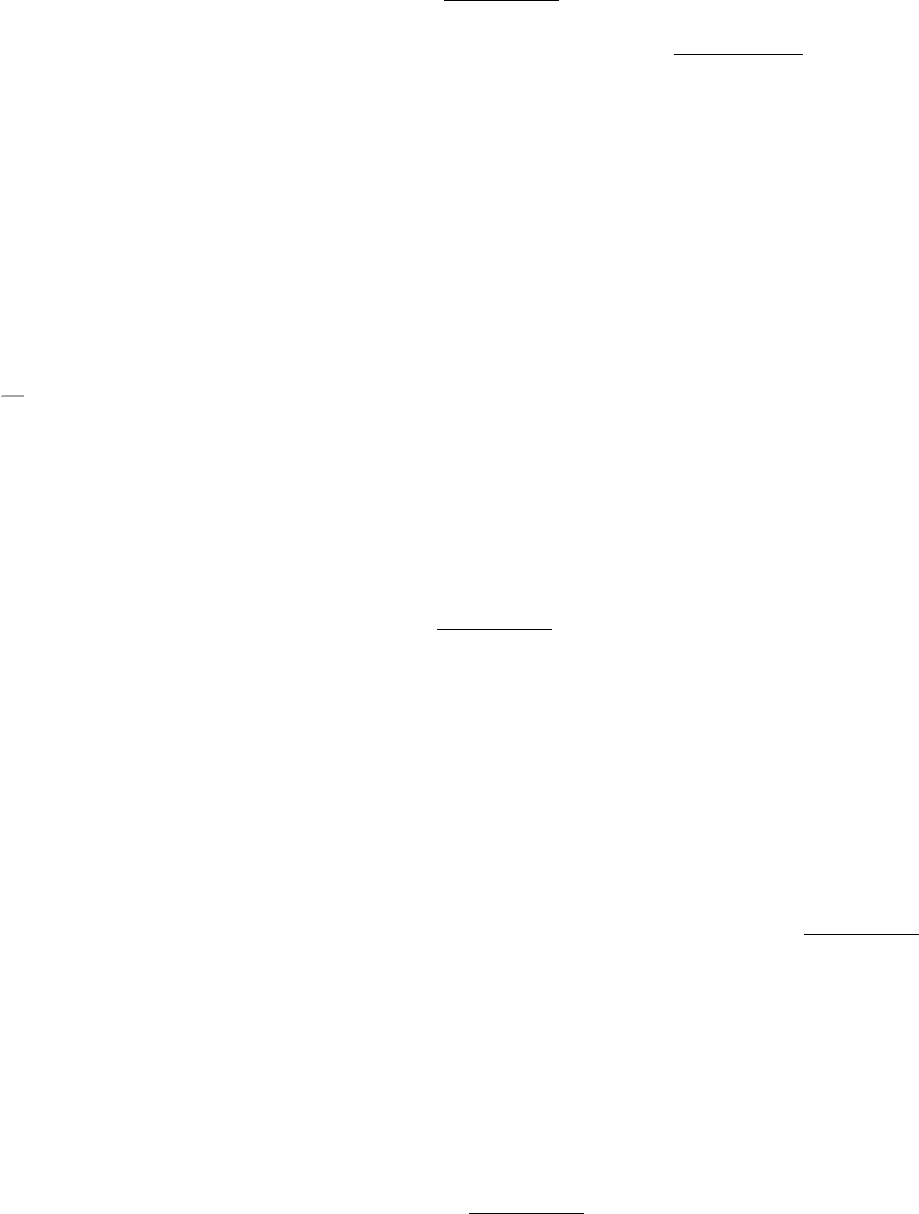
Most aminoacyl-tRNA synthetases contain editing sites in addition to acylation sites. These complementary pairs of sites
function as a double sieve to ensure very high fidelity. In general, the acylation site rejects amino acids that are larger
than the correct one because there is insufficient room for them, whereas the hydrolytic site cleaves activated species that
are smaller than the correct one.
The structure of the complex between threonyl-tRNA synthetase and its substrate reveals that the aminoacylated-CCA
can swing out of the activation site and into the editing site (Figure 29.10). Thus, the aminoacyl-tRNA can be edited
without dissociating from the synthetase. This proofreading, which depends on the conformational flexibility of a short
stretch of polynucleotide sequence, is entirely analogous to that of DNA polymerase (Section 27.2.4). In both cases,
editing without dissociation significantly improves fidelity with only modest costs in time and energy.
A few synthetases achieve high accuracy without editing. For example, tyrosyl-tRNA synthetase has no difficulty
discriminating between tyrosine and phenylalanine; the hydroxyl group on the tyrosine ring enables tyrosine to bind to
the enzyme 10
4
times as strongly as phenylalanine. Proof-reading has been selected in evolution only when fidelity must
be enhanced beyond what can be obtained through an initial binding interaction.
29.2.4. Synthetases Recognize the Anticodon Loops and Acceptor Stems of Transfer
RNA Molecules
How do synthetases choose their tRNA partners? This enormously important step is the point at which "translation"
takes place
at which the correlation between the amino acid and the nucleic acid worlds is made. In a sense, aminoacyl-
tRNA synthetases are the only molecules in biology that "know" the genetic code. Their precise recognition of tRNAs is
as important for high-fidelity protein synthesis as is the accurate selection of amino acids.
A priori, the anticodon of tRNA would seem to be a good identifier because each type of tRNA has a different one.
Indeed, some synthetases recognize their tRNA partners primarily on the basis of their anticodons, although they may
also recognize other aspects of tRNA structure. The most direct evidence comes from the results of crystallographic
studies of complexes formed between synthetases and their cognate tRNAs. Consider, for example, the structure of the
complex between threonyl-tRNA synthetase and tRNA
Thr
(Figure 29.11). As expected, the CCA arm extends into the
zinc-containing activation site, where it is well positioned to accept threonine from threonyl adenylate. The enzyme
interacts extensively not only with the acceptor stem of the tRNA, but also with the anticodon loop. The interactions with
the anticodon loop are particularly revealing. The bases within the sequence CGU of the anticodon each participate in
hydrogen bonds with the enzyme; those in which G and U take part appear to be more important because the C can be
replaced by G or U with no loss of acylation efficiency. The importance of the anticodon bases is further underscored by
studies of tRNA
Met
. Changing the anticodon sequence of this tRNA from CAU to GGU allows tRNA
Met
to be
aminoacylated by threonyl-tRNA synthetase nearly as well as tRNA
Thr
, despite considerable differences in sequence
elsewhere in the structure.
The structure of another complex between a tRNA and an aminoacyl-tRNA synthetase, that of glutaminyl-tRNA
synthetase, again reveals extensive interactions with both the anticodon loop and the acceptor stem (Figure 29.12). In
addition, contacts are made near the "elbow" of the tRNA molecule, particularly with the base pair formed by G in
position 10 and C in position 25 (denoted position 10:25). Reversal of this base pair from G · C to C · G results in a
fourfold decrease in the rate of aminoacylation as well as a fourfold increase in the K
M
value for glutamine. The results
of mutagenesis studies supply further evidence regarding tRNA specificity, even for aminoacyl-tRNA synthetases for
which structures have not yet been determined. For example, E. coli tRNA
Cys
differs from tRNA
Ala
at 40 positions and
contains a C · G base pair at the 3:70 position. When this C · G base pair is changed to the non-Watson-Crick G · U base
pair, tRNA
Cys
is recognized by alanyl-tRNA synthetase as though it were tRNA
Ala
. This finding raised the question
whether a fragment of tRNA suffices for aminoacylation by alanyl-tRNA synthetase. Indeed, a "microhelix" containing
just 24 of the 76 nucleotides of the native tRNA is specifically aminoacylated by the alanyl-tRNA synthetase. This
microhelix contains only the acceptor stem and a hairpin loop (Figure 29.13). Thus, specific aminoacylation is possible
for some synthetases even if the anticodon loop is completely lacking.