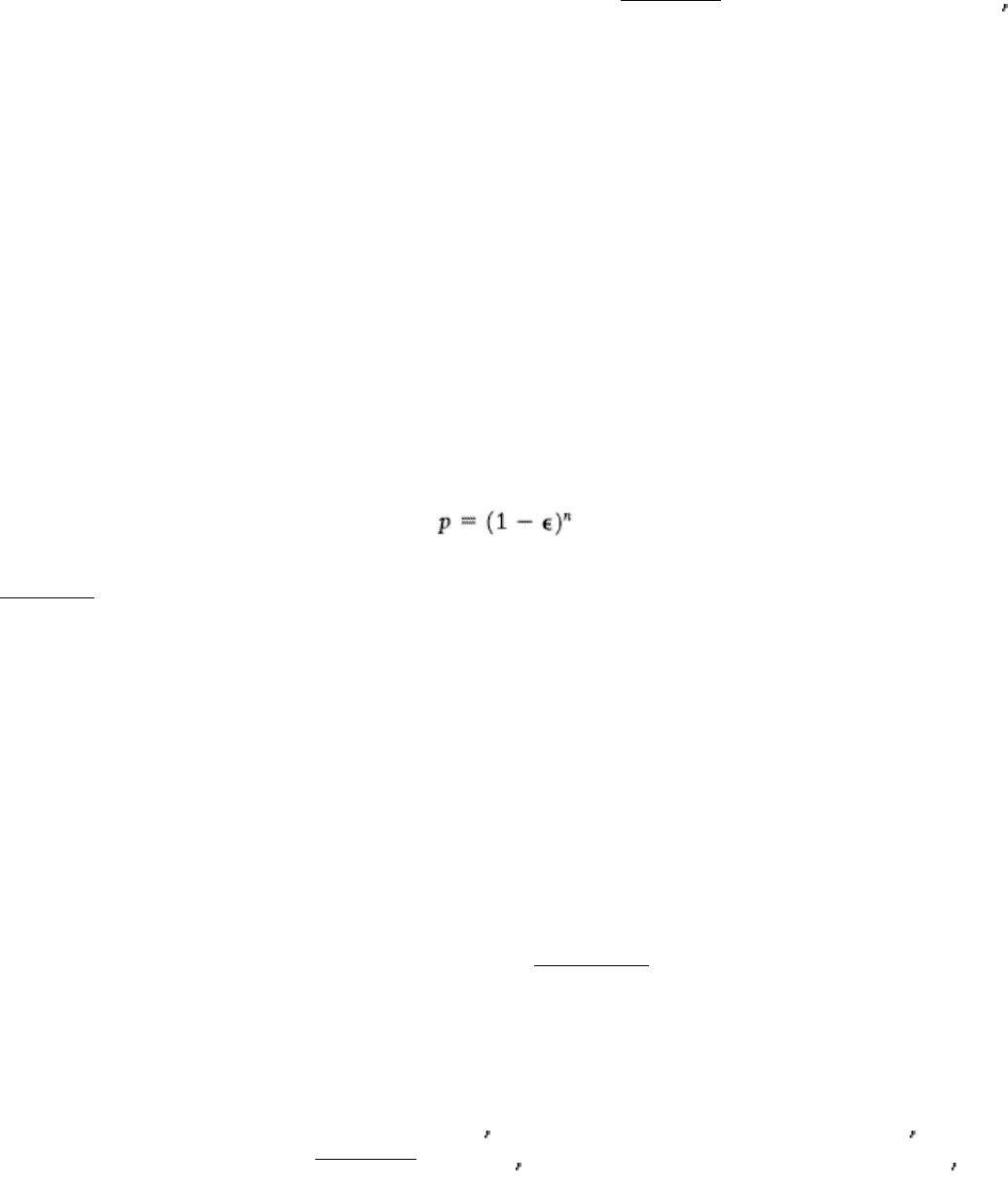
III. Synthesizing the Molecules of Life 29. Protein Synthesis
29.1. Protein Synthesis Requires the Translation of Nucleotide Sequences Into Amino
Acid Sequences
The basics of protein synthesis are the same across all kingdoms of life, attesting to the fact that the protein-synthesis
system arose very early in evolution. A protein is synthesized in the amino-to-carboxyl direction by the sequential
addition of amino acids to the carboxyl end of the growing peptide chain (Figure 29.2). The amino acids arrive at the
growing chain in activated form as aminoacyl-tRNAs, created by joining the carboxyl group of an amino acid to the 3
end of a transfer RNA molecule. The linking of an amino acid to its corresponding tRNA is catalyzed by an aminoacyl-
tRNA synthetase. ATP cleavage drives this activation reaction. For each amino acid, there is usually one activating
enzyme and at least one kind of tRNA.
29.1.1. The Synthesis of Long Proteins Requires a Low Error Frequency
The process of transcription is analogous to copying, word for word, a page from a book. There is no change of alphabet
or vocabulary; so the likelihood of a change in meaning is small. Translating the base sequence of an mRNA molecule
into a sequence of amino acids is similar to translating the page of a book into another language. Translation is a
complex process, entailing many steps and dozens of molecules. The potential for error exists at each step. The
complexity of translation creates a conflict between two requirements: the process must be not only accurate, but also
fast enough to meet a cell's needs. How fast is "fast enough"? In E.coli, translation takes place at a rate of 40 amino acids
per second, a truly impressive speed considering the complexity of the process.
How accurate must protein synthesis be? Let us consider error rates. The probability p of forming a protein with no
errors depends on n, the number of amino acid residues, and ε, the frequency of insertion of a wrong amino acid:
As Table 29.1 shows, an error frequency of 10
-2
would be intolerable, even for quite small proteins. An ε value of 10
-3
would usually lead to the error-free synthesis of a 300-residue protein (~33 kd) but not of a 1000-residue protein (~110
kd). Thus, the error frequency must not exceed approximately 10
-4
to produce the larger proteins effectively. Lower error
frequencies are conceivable; however, except for the largest proteins, they will not dramatically increase the percentage
of proteins with accurate sequences. In addition, such lower error rates are likely to be possible only by a reduction in the
rate of protein synthesis because additional time for proofreading will be required. In fact, the observed values of ε are
close to 10
-
4
. An error frequency of about 10
-4
per amino acid residue was selected in the course of evolution to
accurately produce proteins consisting of as many as 1000 amino acids while maintaining a remarkably rapid rate for
protein synthesis.
29.1.2. Transfer RNA Molecules Have a Common Design
The fidelity of protein synthesis requires the accurate recognition of three-base codons on messenger RNA. Recall that
the genetic code relates each amino acid to a three-letter codon (Section 5.5.1). An amino acid cannot itself recognize a
codon. Consequently, an amino acid is attached to a specific tRNA molecule that can recognize the codon by Watson-
Crick base pairing. Transfer RNA serves as the adapter molecule that binds to a specific codon and brings with it an
amino acid for incorporation into the polypeptide chain.
Robert Holley first determined the base sequence of a tRNA molecule in 1965, as the culmination of 7 years of effort.
Indeed, his study of yeast alanyl-tRNA provided the first complete sequence of any nucleic acid. This adapter molecule
is a single chain of 76 ribonucleotides (Figure 29.3). The 5 terminus is phosphorylated (pG), whereas the 3 terminus has
a free hydroxyl group. The amino acid attachment site is the 3
-hydroxyl group of the adenosine residue at the 3