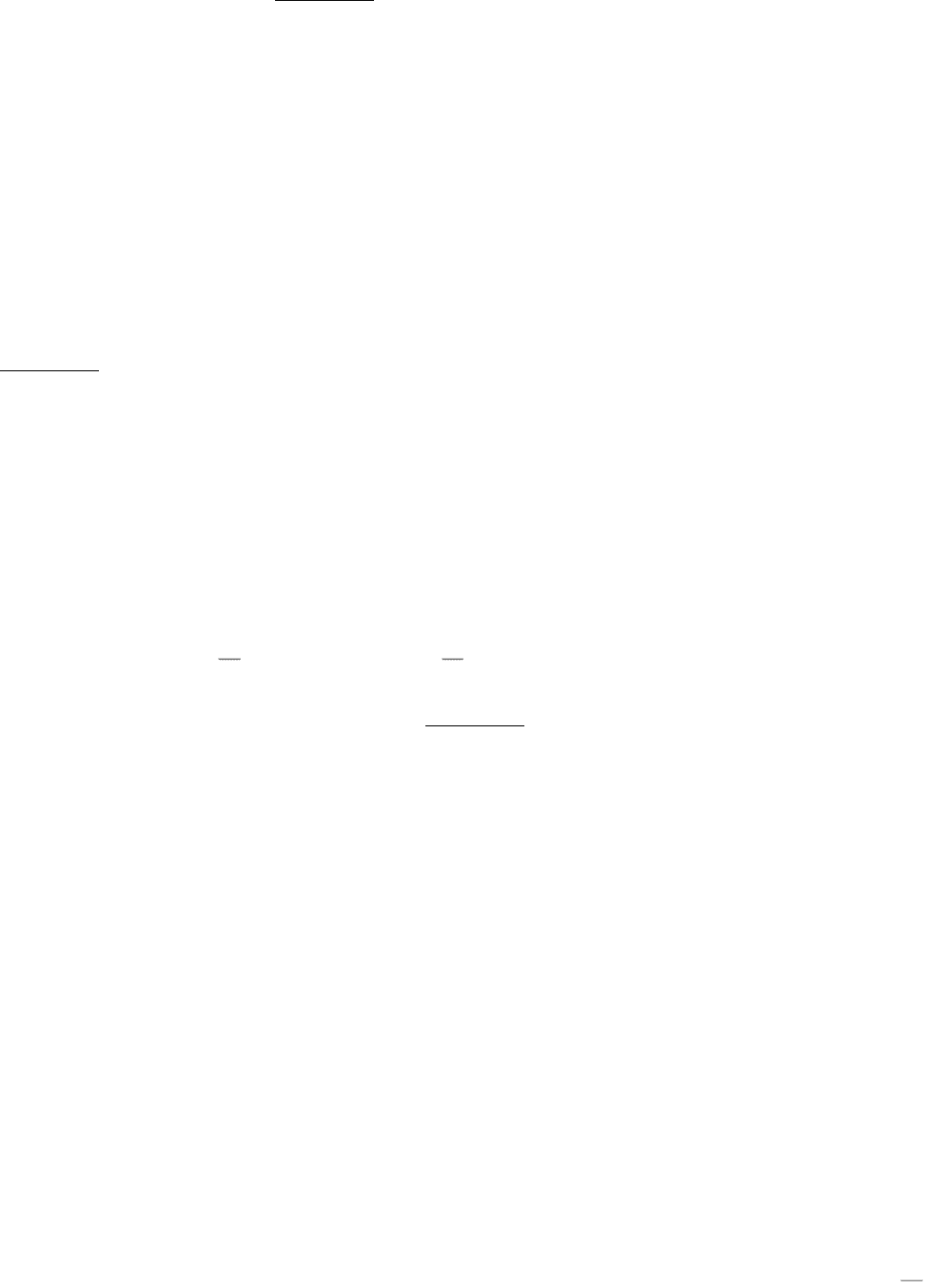
aspartate residue in the ATPase (Figure 13.3).
13.2.1. The Sarcoplasmic Reticulum Ca
2+
ATPase Is an Integral Membrane Protein
We will consider the structural and mechanistic features of these enzymes by examining the Ca
2+
ATPase found in the
sarcoplasmic reticulum (SR Ca
2+
ATPase) of muscle cells. This enzyme, which constitutes 80% of the sarcoplasmic
reticulum membrane protein, plays an important role in muscle contraction, which is triggered by an abrupt rise in the
cytosolic calcium level. Muscle relaxation depends on the rapid removal of Ca
2+
from the cytosol into the sarcoplasmic
reticulum, a specialized compartment for calcium storage, by the SR Ca
2+
ATPase. This pump maintains a Ca
2+
concentration of approximately 0.1 µM in the cytosol compared with 1.5 mM in the sarcoplasmic reticulum.
The SR Ca
2+
ATPase is a single 110-kd polypeptide with a transmembrane domain consisting of 10 α helices. A large
cytoplasmic head piece constitutes nearly half the molecular weight of the protein and consists of three distinct domains
(Figure 13.4). The three cytoplasmic domains of the SR Ca
2+
ATPase have distinct functions. One domain (N) binds the
ATP nucleotide, another (P) accepts the phosphoryl group on its conserved aspartate residue, and the third (A) may serve
as an actuator for the N domain. The relation between these three domains changes significantly on ATP hydrolysis. The
crystal structure in the absence of ATP shows the likely nucleotide-binding site separated by more than 25 Å from the
phosphorylation site, suggesting that the N and P domains move toward one another during the catalytic cycle. This
closure is facilitated by ATP binding and by the binding of Ca
2+
to the membrane-spanning helices.
The results of mechanistic studies of the SR Ca
2+
ATPase and other P-type ATPases have revealed two common
features. First, as we have seen, each protein can be phosphorylated on a specific aspartate residue. For the SR Ca
2+
ATPase, this reaction takes place at Asp 351 only in the presence of relatively high cytosolic concentrations of Ca
2+
.
Second, each pump can interconvert between at least two different conformations, denoted by E
1
and E
2
. Thus, at least
four conformational states
E
1
, E
1
-P, E
2
-P, and E
2
participate in the transport process. From these four states, it is
possible to construct a plausible mechanism of action for these enzymes, although further studies are necessary to
confirm the mechanism and provide more details (Figure 13.5):
1. The postulated reaction cycle begins with the binding of ATP and two Ca
2+
ions to the E
1
state.
2. The enzyme cleaves ATP, transferring the γ-phosphoryl group to the key aspartate residue. Calcium must be bound to
the enzyme for the phosphorylation to take place. Phosphorylation shifts the conformational equilibrium of the ATPase
toward E
2
.
3. The transition from the E
1
to the E
2
state causes the ion-binding sites to "evert" so that the ions can dissociate only to
the luminal side of the membrane.
4. In the E
2
-P state, the enzyme has low affinity for the Ca
2+
ions, so they are released.
5. With the release of Ca
2+
, the phosphoaspartate residue is hydrolyzed, and the phosphate group is released.
6. The enzyme, devoid of a covalently attached phosphoryl group, is not stable in the E
2
form. It everts back to the E
1
form, completing the cycle.
Essentially the same mechanism is employed by the Na
+
-K
+
ATPase. The E
1
state binds three Na
+
ions and transports
them across the membrane and out of the cell as a result of the protein's phosphorylation and transition to the E
2
state.
The three Na
+
ions are released into the extracellular medium. The E
2
state of this enzyme also binds ions namely, two
K
+
ions. These K
+
ions are carried across the membrane in the opposite direction by eversion driven by the hydrolysis of
the phosphoaspartate residue and are released into the cytosol.