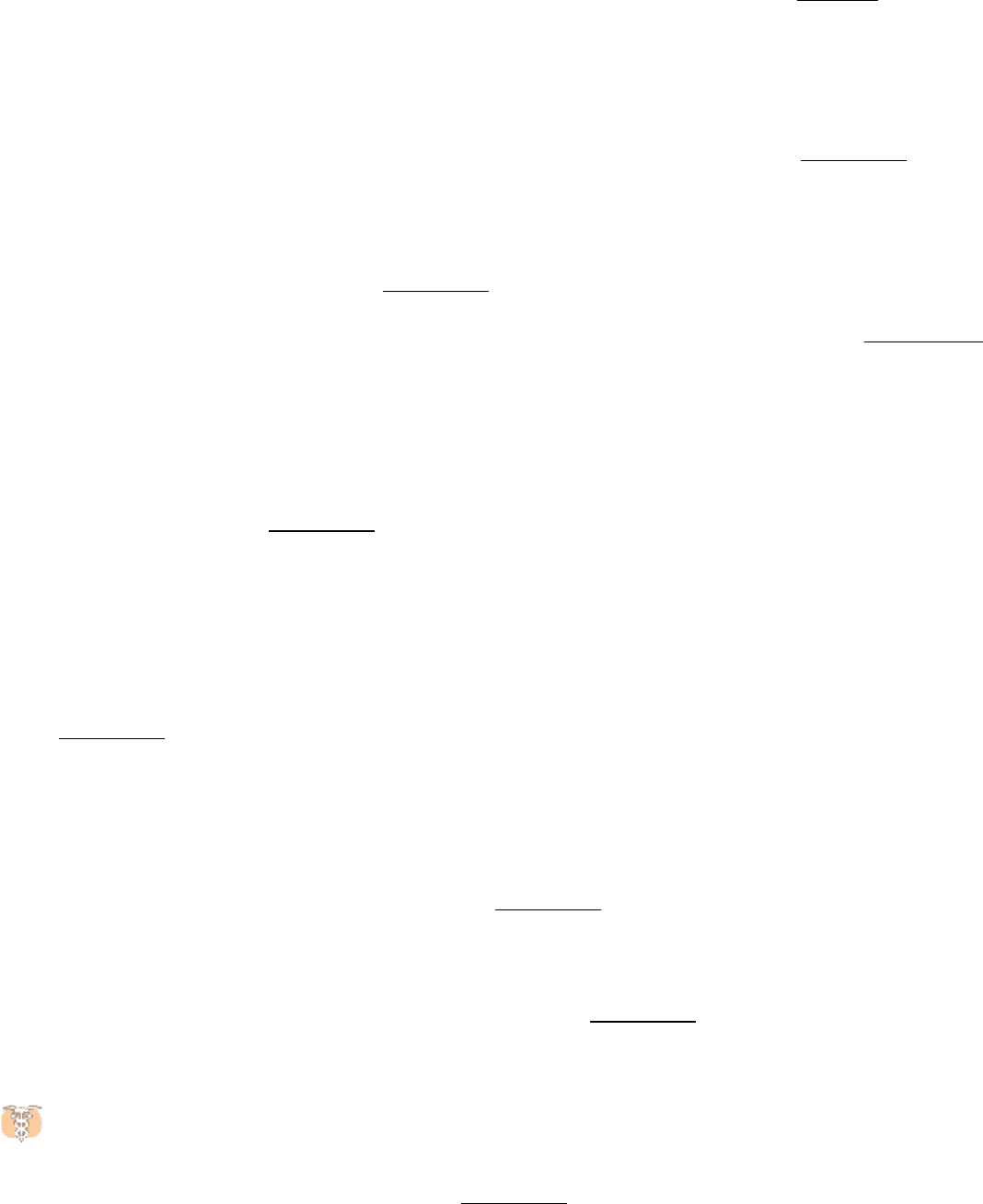
Although membrane proteins are more difficult to purify and crystallize than are water-soluble proteins, researchers
using x-ray crystallographic or electron microscopic methods have determined the three-dimensional structures of more
than 20 such proteins at sufficiently high resolution to discern the molecular details. As noted in Chapter 3, the structures
of membrane proteins differ from those of soluble proteins with regard to the distribution of hydrophobic and
hydrophilic groups. We will consider the structures of three membrane proteins in some detail.
Proteins Can Span the Membrane with Alpha Helices.
The first membrane protein that we consider is the archaeal protein bacteriorhodopsin, shown in Figure 12.18. This
protein acts in energy transduction by using light energy to transport protons from inside the cell to outside. The proton
gradient generated in this way is used to form ATP. Bacteriorhodopsin is built almost entirely of α helices; seven closely
packed α helices, arranged almost perpendicularly to the plane of the membrane, span its 45-Å width. Examination of
the primary structure of bacteriorhodopsin reveals that most of the amino acids in these membrane-spanning α helices
are nonpolar and only a very few are charged (Figure 12.19). This distribution of nonpolar amino acids is sensible
because these residues are either in contact with the hydrocarbon core of the membrane or with one another. Membrane-
spanning α helices are the most common structural motif in membrane proteins. As will be discussed in Section 12.5.4,
such regions can often be detected from amino acid sequence information alone.
A Channel Protein Can Be Formed from Beta Strands.
Porin, a protein from the outer membranes of bacteria such as E. coli and Rhodo-bacter capsulatus, represents a class of
membrane proteins with a completely different type of structure. Structures of this type are built from β strands and
contain essentially no α helices (Figure 12.20).
The arrangement of β strands is quite simple: each strand is hydrogen bonded to its neighbor in an antiparallel
arrangement, forming a single β sheet. The β sheet curls up to form a hollow cylinder that functions as the active unit. As
its name suggests, porin forms pores, or channels, in the membranes. A pore runs through the center of each cylinder-like
protein. The outside surface of porin is appropriately nonpolar, given that it interacts with the hydrocarbon core of the
membrane. In contrast, the inside of the channel is quite hydrophilic and is filled with water. This arrangement of
nonpolar and polar surfaces is accomplished by the alternation of hydrophobic and hydrophilic amino acids along each β
strand (Figure 12.21).
Embedding Part of a Protein in a Membrane Can Link It to the Membrane Surface.
The structure of the membrane-bound enzyme prostaglandin H
2
synthase-1 reveals a rather different role for α helices in
protein-membrane associations. This enzyme catalyzes the conversion of arachidonic acid into prostaglandin H
2
in two
steps: a cyclooxygenase reaction and a peroxidase reaction (Figure 12.22). Prostaglandin H
2
promotes inflammation and
modulates gastric acid secretion. The enzyme that produces prostaglandin H
2
is a homodimer with a rather complicated
structure consisting primarily of α helices. Unlike bacteriorhodopsin, this protein is not largely embedded in the
membrane but, instead, lies along the outer surface of the membrane firmly bound by a set of α helices with hydrophobic
surfaces that extend from the bottom of the protein into the membrane (Figure 12.23). This linkage is sufficiently strong
that only the action of detergents can release the protein from the membrane. Thus, this enzyme is classified as an
integral membrane protein, although it is not a membrane-spanning protein.
The localization of prostaglandin H
2
synthase-l in the membrane is crucial to its function. The substrate for this
enzyme, arachidonic acid, is a hydrophobic molecule generated by the hydrolysis of membrane lipids.
Arachidonic acid reaches the active site of the enzyme from the membrane without entering an aqueous environment by
traveling through a hydrophobic channel in the protein (Figure 12.24). Indeed, nearly all of us have experienced the
importance of this channel: drugs such as aspirin and ibuprofen block the channel and prevent prostaglandin synthesis by
inhibiting the cyclooxygenase activity of the synthase. In the case of aspirin, the drug acts through the transfer of an