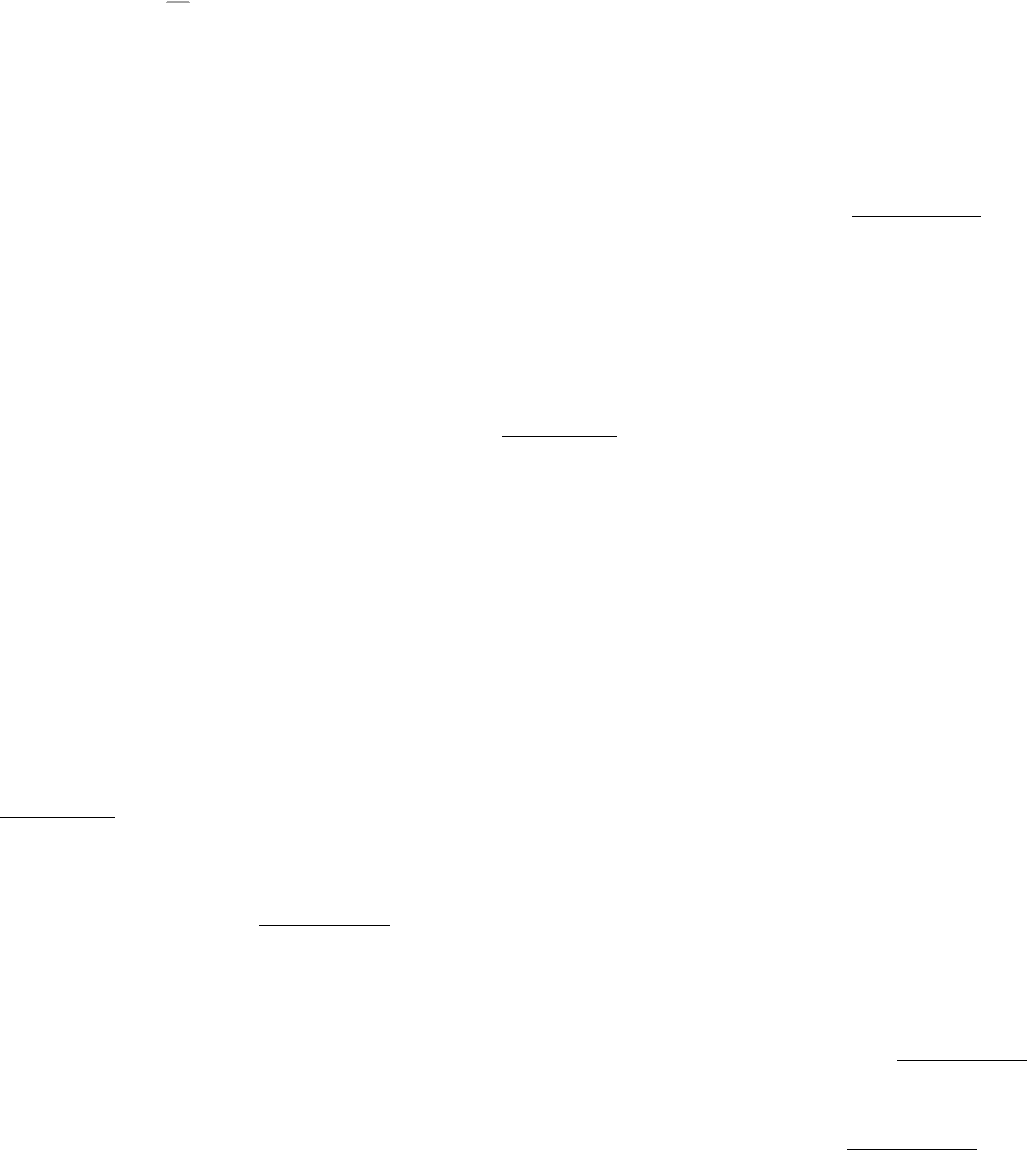
transduction cascades.
15.2.1. Inositol 1,4,5-trisphosphate Opens Channels to Release Calcium Ions from
Intracellular Stores
What are the biochemical effects of the second messenger inositol 1,4,5-trisphosphate? These effects were delineated by
microinjecting IP
3
molecules into cells or by allowing IP
3
molecules to diffuse into cells whose plasma membranes had
been made permeable. Michael Berridge and coworkers found that IP
3
causes the rapid release of Ca
2
+
from
intracellular stores
the endoplasmic reticulum and, in smooth muscle cells, the sarcoplasmic reticulum. The elevated
level of Ca
2
+
in the cytosol then triggers processes such as smooth muscle contraction, glycogen breakdown, and
vesicle release. In Xenopus oocytes, the injection of IP
3
suffices to activate many of the early events of fertilization.
Inositol 1,4,5-trisphosphate is able to increase Ca
2+
concentration by associating with a membrane protein called the IP
3
-gated channel or IP
3
receptor. This receptor, which is composed of four large, identical subunits, forms an ion channel.
The ion-conducting channel itself is likely similar to the structurally characterized K
+
channel (Section 13.5.6). At least
three molecules of IP
3
must bind to sites on the cytosolic side of the membrane protein to open the channel and release
Ca
2+
. The highly cooperative opening of calcium channels by nanomolar concentrations of IP
3
enables cells to detect
and amplify very small changes in the concentration of this messenger. The cooperativity of IP
3
binding and channel
opening is another example of the role of allosteric interactions.
How is the IP
3
-initiated signal turned off? Inositol 1,4,5-trisphosphate is a short-lived messenger because it is rapidly
converted into derivatives that do not open the channel (Figure 15.14). Its lifetime in most cells is less than a few
seconds. Inositol 1,4,5-trisphosphate can be degraded to inositol by the sequential action of phosphatases or it can be
phosphorylated to inositol 1,3,4,5-tetrakisphosphate, which is then converted into inositol by an alternative route.
Lithium ion, widely used to treat bipolar affective disorder, may act by inhibiting the recycling of inositol 1,3,4-
trisphosphate.
15.2.2. Diacylglycerol Activates Protein Kinase C, Which Phosphorylates Many Target
Proteins
Diacylglycerol, the other molecule formed by the receptor-triggered hydrolysis of PIP
2
, also is a second messenger and
it, too, activates a wide array of targets. We will examine how diacylglycerol activates protein kinase C (PKC), a protein
kinase that phosphorylates serine and threonine residues in many target proteins. The amino acid sequences and the
results of three-dimensional structural studies of protein kinase C isozymes reveal an elegant modular architecture
(Figure 15.15). The α, β, and γ isozymes of protein kinase C have in common a structurally similar catalytic domain,
homologous to that in PKA, at the carboxyl terminus. Adjacent to the catalytic domain is a C2 domain that is related to
the C2 domain of phospholipase C and that interacts with membrane phospholipids. On the other side of the C2 domain
is a pair of C1 domains, each organized around two bound zinc ions. These two domains, particularly the second (C1B)
domain, bind diacylglycerol (Figure 15.16A). Finally, at the amino terminus is a sequence of the form -A-R-K-G-A-L-R-
Q-K-. This sequence is striking because the consensus sequence for PKC substrates is X-R-X-X-(S,T)-Hyd-R-X in
which Hyd refers to a large, hydrophobic residue. This sequence from PKC is referred to as a pseudosubstrate sequence
because it resembles the substrate sequence except that it has an alanine residue in place of the serine or threonine
residue; so it cannot be phosphorylated. Recall that an analogous pseudosubstrate sequence is present in the regulatory
chain of PKA and that it prevents activity by occluding the active site in the R
2
C
2
tetramer of PKA (Section 10.4.2).
Similarly, the pseudosubstrate sequence of PKC binds to that enzyme's active site and prevents substrate binding.
With this structure in mind, we can now understand how PKC is activated on PIP
2
hydrolysis (Figure 15.16B). Before
activation, PKC is free in solution. On PIP
2
hydrolysis in the membrane by phospholipase C, the C1B domain of PKC