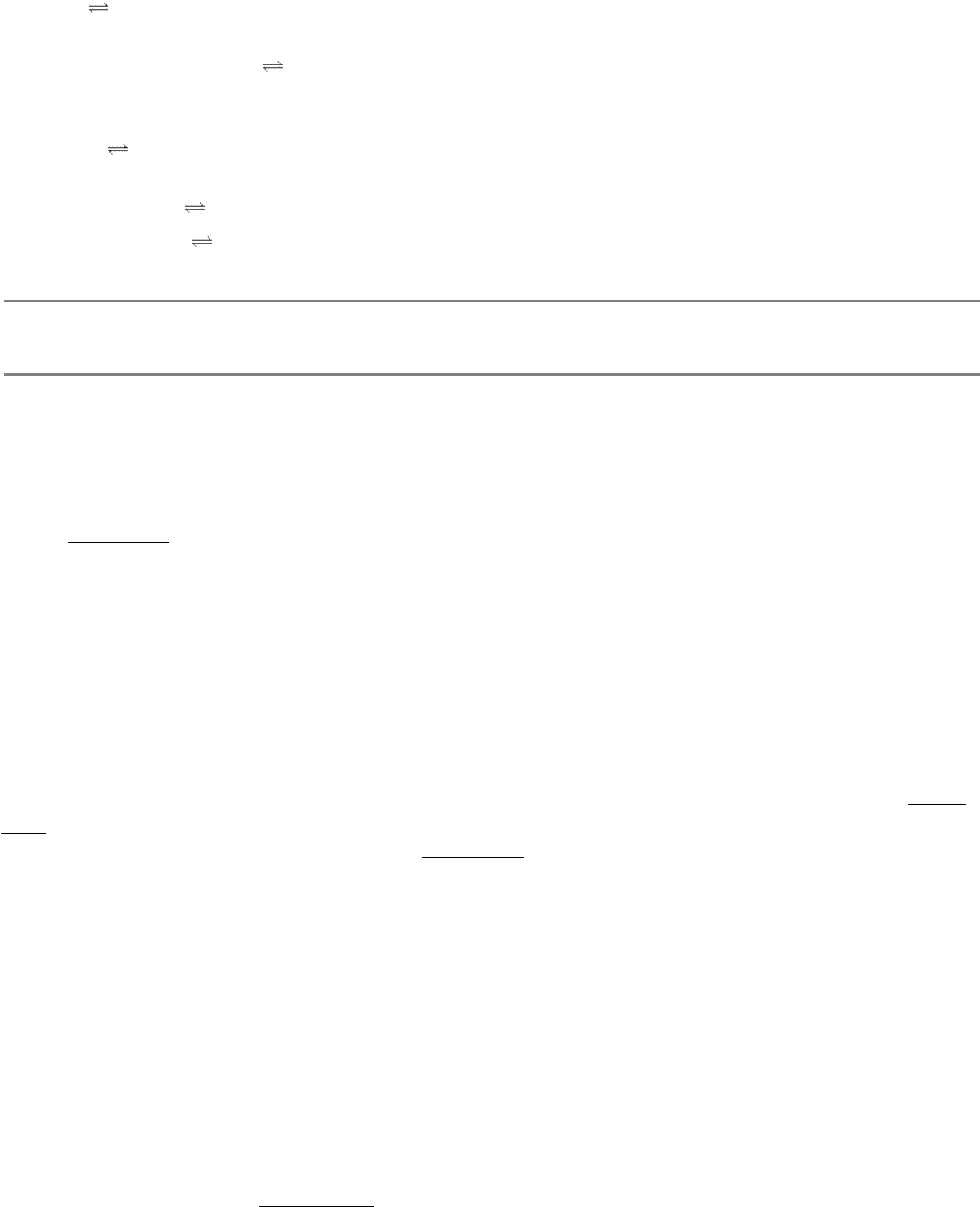
4
α-Ketoglutarate + NAD
+
+
CoA succinyl CoA + CO
2
+ NADH
α-Ketoglutarate dehydrogenase
complex
Lipoic acid, FAD,
TPP
d + e -7.2 -30.1
5 Succinyl CoA + P
i
+ GDP
succinate + GTP + CoA
Succinyl CoA synthetase f -0.8 -3.3
6 Succinate + FAD (enzyme-
bound)
fumarate +
FADH
2
(enzyme-bound)
Succinate dehydrogenase FAD, Fe-S e ˜0 0
7 Fumarate + H
2
O l-malate Furmarase c -0.9 -3.8
8
l-Malate + NAD
+
oxaloacetate + NADH + H
+
Malate dehydrogenase e +7.1 +29.7
*
Reaction type: (a) condensation; (b) dehydration; (c) hydration; (d) decarboxylation; (e) oxidation; (f) substrate-level
phosphorylation.
II. Transducing and Storing Energy 17. The Citric Acid Cycle
17.2. Entry to the Citric Acid Cycle and Metabolism Through It Are Controlled
The citric acid cycle is the final common pathway for the aerobic oxidation of fuel molecules. Moreover, as we will see
shortly (Section 17.3) and repeatedly elsewhere in our study of biochemistry, the cycle is an important source of building
blocks for a host of important biomolecules. As befits its role as the metabolic hub of the cell, entry into the cycle and
the rate of the cycle itself are controlled at several stages.
17.2.1. The Pyruvate Dehydrogenase Complex Is Regulated Allosterically and by
Reversible Phosphorylation
As we saw earlier, glucose can be formed from pyruvate (Section 16.3). However, the formation of acetyl CoA from
pyruvate is an irreversible step in animals and thus they are unable to convert acetyl CoA back into glucose. The
oxidative decarboxylation of pyruvate to acetyl CoA commits the carbon atoms of glucose to two principal fates:
oxidation to CO
2
by the citric acid cycle, with the concomitant generation of energy, or incorporation into lipid (Figure
17.16). As expected of an enzyme at a critical branch point in metabolism, the activity of the pyruvate dehydrogenase
complex is stringently controlled by several means (Figure 17.17). High concentrations of reaction products of the
complex inhibit the reaction: acetyl CoA inhibits the transacetylase component (E
2
), whereas NADH inhibits the
dihydrolipoyl dehydrogenase (E
3
). However, the key means of regulation in eukaryotes is covalent modification of the
pyruvate dehydrogenase component. Phos-phorylation of the pyruvate dehydrogenase component (E
1
) by a specific
kinase switches off the activity of the complex. Deactivation is reversed by the action of a specific phosphatase. The site
of phosphorylation is the transacetylase component (E
2
), again highlighting the structural and mechanistic importance of
this core. Increasing the NADH/NAD
+
, acetyl CoA/CoA, or ATP/ADP ratio promotes phosphorylation and, hence,
deactivation of the complex. In other words, high concentrations of immediate (acetyl CoA and NADH) and ultimate
(ATP) products inhibit the activity. Thus, pyruvate dehydrogenase is switched off when the energy charge is high and
biosynthetic intermediates are abundant. On the other hand, pyruvate as well as ADP (a signal of low energy charge)
activate the dehydrogenase by inhibiting the kinase.
In contrast, α
1
-adrenergic agonists and hormones such as vasopressin stimulate pyruvate dehydrogenase by triggering a
rise in the cytosolic Ca
2+
level (Section 15.3.2), which in turn elevates the mitochondrial Ca
2+
level. The rise in
mitochondrial Ca
2+
activates the pyruvate dehydrogenase complex by stimulating the phosphatase. Insulin also
accelerates the conversion of pyruvate into acetyl CoA by stimulating the dephosphorylation of the complex. In turn,
glucose is funneled into pyruvate.