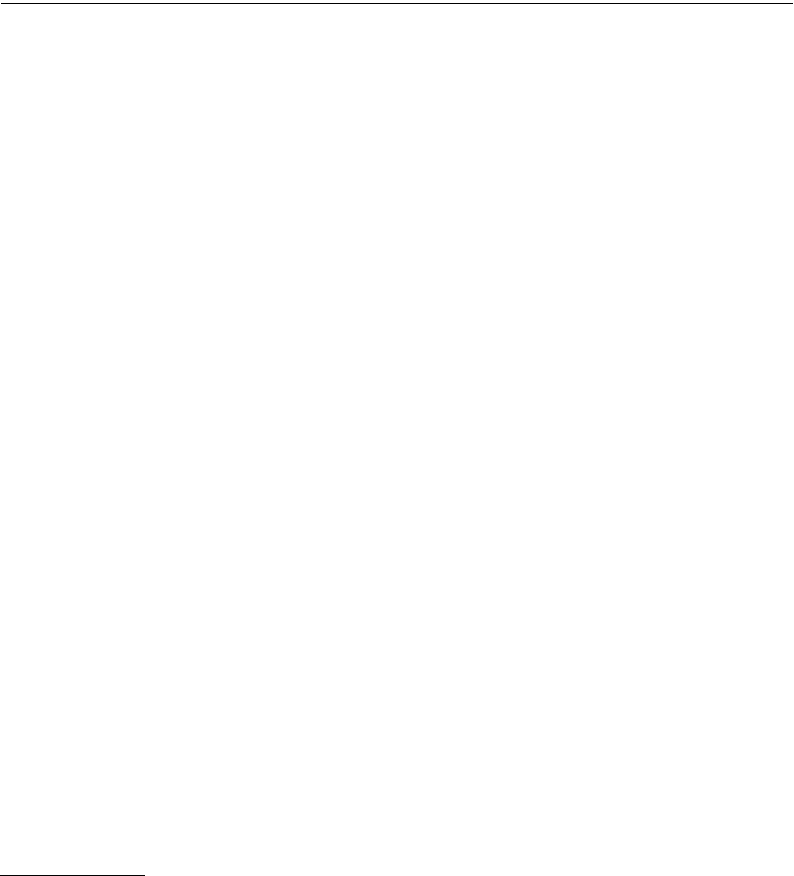
© 1999 by CRC Press LLC
When the asperities were separated vertically by large distances (the first simulation geometry), the shear
flow was accompanied by a structuring of the liquid with no distortions of the metal surfaces. In contrast,
for the overlapping and near-overlapping cases, shearing resulted in pressurization of the liquid in the
interasperity region, accompanied by a significant increase in effective viscosity. This resulted in plastic
deformations of the gold asperities.
It is clear, based on the discussion in this section, that fluids confined to areas of atomic-scale
dimensions do not necessarily behave like liquids on the macroscopic scale. In fact, depending on the
condition, they may often behave more like solids in terms of structure and flow. This presents a new
set of concerns for lubricating moving parts at the nanometer scale. However, with the aid of simulations
like the ones mentioned here, plus experimental studies using techniques such as the SFA, general
properties of nanometer-scale fluids are being characterized effectively with profound precision. This, in
turn, will allow scientists and engineers to design new materials and interfaces that will have specific
interactions with confined lubricants, effectively controlling friction (and wear) at the atomic scale.
11.5 Friction
Friction at sliding solid interfaces results from the conversion of the work of sliding into some other,
less-ordered, form. For strongly adhering systems, the work of sliding may be converted into damage
within the bulk. As adhesive forces are decreased between the solid surfaces, the conversion of work
changes to mechanical damage at or near the surface, leading to, for example, the formation of wear
debris and transfer films (Singer, 1991; Singer et al., 1991). For still weaker adhesive forces, friction can
occur through the conversion of work to heat at the interface with no permanent damage to the surface.
The latter regime is the topic of this section.
While the thermodynamic principles of the conversion of work to heat are well known, many of the
detailed mechanisms at sliding interfaces are just beginning to be understood. This understanding is the
by-product of new scientific instrumentation, such as the SFA and the AFM, analytic theories, and
simulations. A detailed understanding of these processes is important for producing interfaces with
specific friction (and wear) properties.
Atomic-scale friction has been investigated using MD simulations for systems composed of a variety
of materials, in a number of geometries. For instance, the atomic-scale friction and wear of diamond
surfaces, both atomically flat and rough, was examined using MD by Harrison et al. (1992c, 1993a,b,c).
In addition, atomic-scale friction between monolayers composed of alkane chains bound to rigid sub-
strates (Glosli and McClelland, 1993), between perfluorocarboxylic acid and hydrocarboxylic LB mono-
layers (Koike and Yoneya, 1996), between contacting Cu surfaces (Hammerberg et al., 1995; Sorensen
et al., 1996), between a silicon tip and a silicon substrate (Landman et al., 1989a,b), and between con-
tacting diamond surfaces in the presence of third-body molecules (Perry and Harrison, 1997) have all
been examined using MD simulations.
Molecular dynamics simulations have also provided the first insights into tribochemical reactions that
might occur when two diamond surfaces are in sliding contact (Harrison and Brenner, 1994).
11.5.1 Solid Lubrication
Harrison et al. (1992c, 1993a,b,c) examined the atomic-scale phenomena that occurred when two (111)
diamond surfaces were placed in sliding contact. The effects of crystallographic sliding direction, tem-
perature, sliding speed, applied normal load, and nature of the surface on atomic-scale friction were
examined. The frictional properties of hydrogen-terminated diamond surfaces were compared with the
frictional properties of alkyl-terminated diamond surfaces to elucidate the effects of surface condition
on atomic-scale friction. The alkyl-terminated systems were composed of two diamond lattices, config-
ured so that their (111) surfaces were in contact (Figures 11.32a to d). One eighth of the hydrogen atoms
on the upper surfaces were then randomly replaced with methyl (Figure 11.32b), ethyl (Figure 11.32c),
or n-propyl (Figure 11.32d) groups.