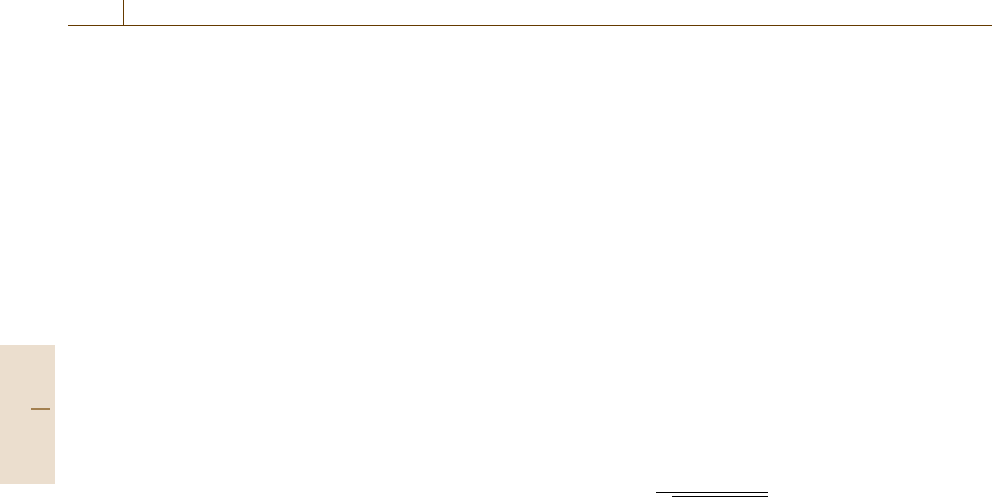
518 Part C Materials Properties Measurement
times and thus a very high speed potential of electronic
circuits.
9.4.4 I–V Breakdown Mechanisms
Electrical breakdown in semiconductors is character-
ized in terms of the electrical field strength (unit
kV/cm) in regions of the I–V curve, where the cur-
rent increases to a much higher extent than for slightly
reduced field strength below. Electrical breakdown, in-
dicated by a steep current increase versus voltage, is
mainly related to two mechanisms: impact ionization
(avalanche) breakdown and tunneling (internal field
emission). Both mechanisms can be distinguished by
investigating the temperature behavior of the current-
voltage (I–V ) curves. For a certain constant voltage
in the breakdown region a positive temperature co-
efficient of the current is indicative for tunneling
breakdown, while a negative temperature coefficient
indicates impact ionization breakdown. Both mecha-
nisms are discussed in more detail in the following two
subsections.
Impact Ionization Breakdown Field Strength
Impact ionization is a bulk controlled current flow
mechanism induced by a carrier multiplying band–band
process. Carriers are accelerated by an high electrical
field, that they can induce electron–hole pair ionization
due to their high energy. The resulting additional elec-
trons and holes are accelerated in opposite directions,
until they gain and overcome their specific ionizing
energy (kT); thus, the whole process produces an
avalanche-like increase of the current after an only
small voltage increase. Impact ionization breakdown
can be concluded from a negative temperature coef-
ficient of the current at a high fixed reverse voltage.
Increasing temperature causes more lattice vibrations,
which hinder carriers, to gain directed acceleration; thus
higher electrical fields are needed at elevated temper-
atures, to produce the same current. Small effective
masses ease the ionization process due to higher car-
rier mobility in the accelerating field. Impact ionization
is a band–band pair generation process, thus the break
down electric field strength increases with increasing
bandgap of the semiconductor. The avalanche process is
described by the ionization rates α
n
and α
h
(unit 1/cm)
for electron or hole induced electron–hole pairs, respec-
tively. The ionization rates α
n,h
depend largely on the
electrical field strength E
α(E) =α
∞
e
[−(E
0
/E)
m
]
(9.57)
with α
∞
, E
0
,andm being a temperature dependent
material constant. Graphs of ionization rates of several
semiconductors can be found in [9.49] ranging up to
values of α
n,h
of 10
5
/cm.
Tunneling (Internal Field Emission)
Breakdown Field Strength
Tunneling or internal field emission is a quantum
mechanical process, where carriers penetrate through
thin energy barriers when the barrier thicknesses ap-
proach the value of the de Broglie wavelength (a few
nm). During the quantum mechanical tunneling pro-
cess their energy is conserved. Carrier tunneling is
a barrier-controlled current flow mechanism. Tunneling
is observed in highly doped pn junctions, where narrow
barriers are favored by the high doping and the resulting
small space charge regions. The de Broglie wavelength
λ
e,h
is given by
λ
e,h
=
h
2 ∗m
0
m
e,h
E
(9.58)
with m
0
denoting the free electron mass, m
e,h
the ef-
fective mass of electrons or holes, and E the energy of
the carrier. For, e.g. InP with an electron effective mass
of 0.07 and an electron energy of kT(T = 300 K)λ
e
amounts to 29 nm, while for E = 1eV, λ
e
reduces
to 4.64 nm.
The barrier to be penetrated by the carrier should be
narrower than de Broglie’s wavelength for the relevant
carrier’s energy. The probability for tunneling increases
considerably for electrical field strength in the range
of 10
6
V/cm, i. e. 1 (e)V/10 nm, where the effective bar-
rier width for band–band tunneling is reduced to 10 nm,
being in the range of the aforementioned de Broglie
wavelength. For this nonthermal process, the thermal
energy of the carrier (electron) is most often neglected.
Temperature Dependent Reverse Current
I–V Analysis
According to the explanations given in the two sub
sections before, the dominating mechanism for break-
down in pn-diodes or transistors can be identified by
measuring the reverse I–V characteristics for various
temperatures. A recommended temperature range ex-
tends, e.g. from 0 to 80
◦
C in steps of 20
◦
C, which can
be controlled by Peltier cooling or heating. Much wider
temperature ranges can be achieved in cryostats, where
electrical heating in conjunction with liquid nitrogen
cooling can provide a temperature range between 77
and 420 K. Because generation currents in pn-junctions
are increasing proportional to the intrinsic concentration
Part C 9.4