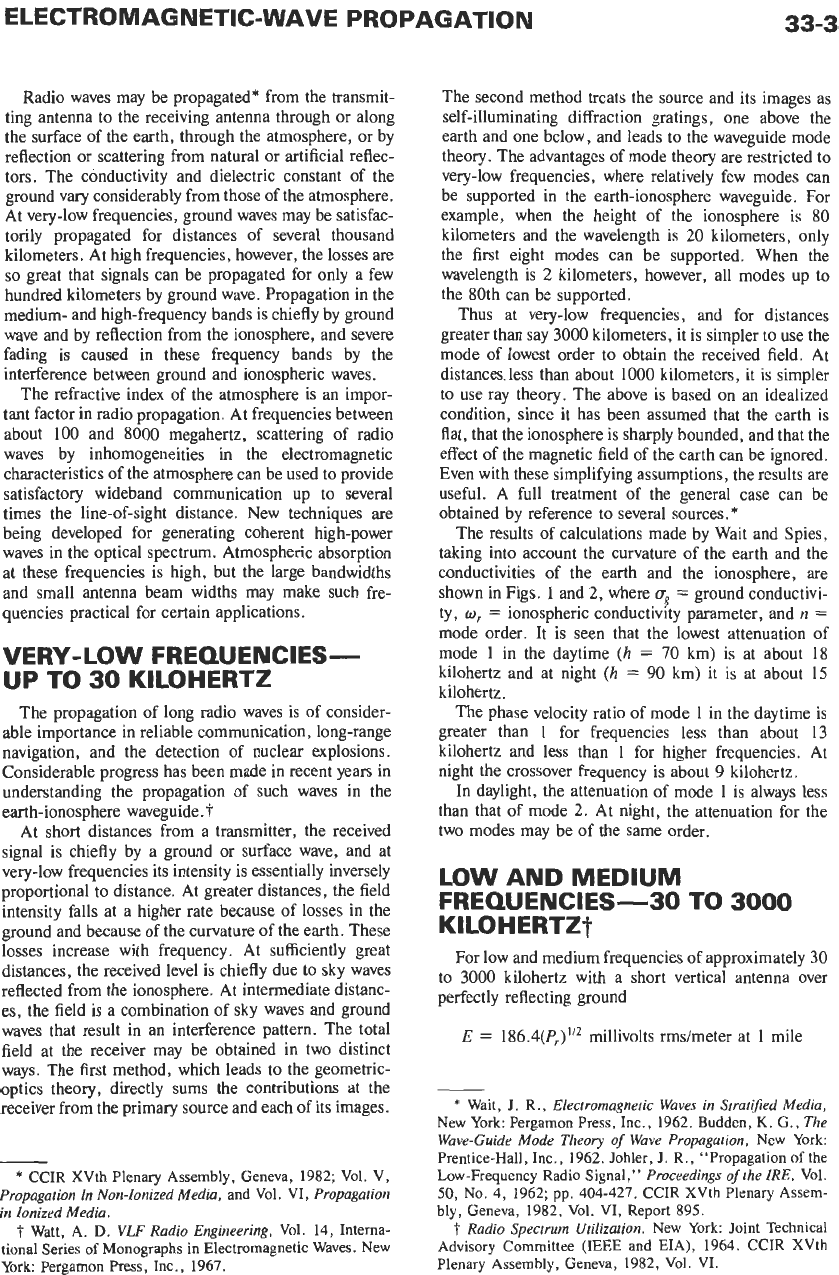
ELECTROMAGNETIC-WAVE PROPAGATION
33-3
Radio waves may be propagated* from the transmit-
ting antenna to the receiving antenna through or along
the surface of the earth, through the atmosphere, or by
reflection or scattering from natural or artificial reflec-
tors. The conductivity and dielectric constant of the
ground vary considerably from those of the atmosphere.
At very-low frequencies, ground waves may be satisfac-
torily propagated for distances of several thousand
kilometers. At high frequencies, however, the losses are
so
great that signals can be propagated for only a few
hundred kilometers by ground wave. Propagation in the
medium- and high-frequency bands is chiefly by ground
wave and by reflection from the ionosphere, and severe
fading is caused in these frequency bands by the
interference between ground and ionospheric waves.
The refractive index of the atmosphere is an impor-
tant factor in radio propagation. At frequencies between
about
100
and
8000
megahertz, scattering of radio
waves by inhomogeneities in the electromagnetic
characteristics of the atmosphere can be used to provide
satisfactory wideband communication up to several
times the line-of-sight distance. New techniques are
being developed for generating coherent high-power
waves in the optical spectrum. Atmospheric absorption
at these frequencies is high, but the large bandwidths
and small antenna beam widths may make such fre-
quencies practical for certain applications.
VERY-LOW FREQUENCIES-
UP
TO
30
KILOHERTZ
The propagation of long radio waves is of consider-
able importance in reliable communication, long-range
navigation, and the detection
of
nuclear explosions.
Considerable progress has been made in recent years in
understanding the propagation of such waves in the
earth-ionosphere waveguide.?
At short distances from a transmitter, the received
signal is chiefly by a ground or surface wave, and at
very-low frequencies its intensity is essentially inversely
proportional to distance. At greater distances, the field
intensity falls at a higher rate because of losses in the
ground and because of the curvature of the earth. These
losses increase with frequency. At sufficiently great
distances, the received level is chiefly due to sky waves
reflected from the ionosphere. At intermediate distanc-
es, the field is a combination of sky waves and ground
waves that result in an interference pattern. The total
field at the receiver may be obtained in two distinct
ways.
The
first method, which leads to the geometric-
optics theory, directly sums the contributions at the
receiver from the primary source and each of its images.
-
*
CCIR XVth Plenary Assembly, Geneva, 1982; Vol. V,
Propagation in Non-Ionized Media,
and Vol. VI,
Propagation
in
Ionized Media.
f
Watt,
A.
D.
VLF
Radio Engineering,
Vol. 14, Interna-
tional Series
of
Monographs in Electromagnetic Waves. New
York Pergamon Press, Inc., 1967.
The second method treats the source and its images as
self-illuminating diffraction gratings, one above the
earth and one below, and leads
to
the waveguide mode
theory. The advantages of mode theory are restricted to
very-low frequencies, where relatively few modes can
be supported in the earth-ionosphere waveguide. For
example, when the height of the ionosphere is 80
kilometers and the wavelength is
20
kilometers, only
the first eight modes can be supported. When the
wavelength is
2
kilometers, however, all modes up to
the 80th can be supported.
Thus at very-low frequencies, and for distances
greater than say
3000
kilometers, it
is
simpler to use the
mode of lowest order to obtain the received field. At
distances.less than about
1000
kilometers, it is simpler
to use ray theory. The above is based on an idealized
condition, since it has been assumed that the earth is
flat, that the ionosphere is sharply bounded, and that the
effect of the magnetic field of the earth can be ignored.
Even with these simplifying assumptions, the results are
useful. A full treatment
of
the general case can be
obtained by reference to several sources.
*
The results of calculations made by Wait and Spies,
taking into account the curvature of the earth and the
conductivities of the earth and the ionosphere, are
shown in Figs.
1
and
2,
where
ug
=
ground conductivi-
ty,
w,
=
ionospheric conductivity parameter, and
n
=
mode order. It is seen that the lowest attenuation of
mode
1
in the daytime
(h
=
70
km) is at about 18
kilohertz and at night
(h
=
90
km) it
is
at about
15
kilohertz.
The phase velocity ratio of mode
1
in the daytime is
greater than
1
for frequencies less than about
13
kilohertz and less than
1
for higher frequencies. At
night the crossover frequency
is
about
9
kilohertz.
In daylight, the attenuation
of
mode
1
is always less
than that of mode
2.
At night, the attenuation for the
two modes may be of the same order.
LOW AND MEDIUM
KILOHERTZT
FREQUENCIES-30 TO
3000
For low and medium frequencies of approximately
30
to
3000
kilohertz with a short vertical antenna over
perfectly reflecting ground
E
=
186.4(P,)”2
millivolts rmdmeter at
1
mile
*
Wait,
J.
R.,
Electromugneric Waves in Srrutified Media,
New York: Pergamon Press, Inc., 1962. Budden,
K.
G.,
The
Wave-Guide Mode Theory
of
Wave Propagation,
New York:
Prentice-Hall, Inc., 1962. Johler,
J.
R., “Propagation
of
the
Low-Frequency Radio Signal,”
Proceedings
of
rhe IRE,
Vol.
50,
No. 4, 1962; pp. 404-427. CCIR XVth Plenary
Assem-
bly, Geneva, 1982, Vol. VI, Report 895.
f
Radio Spectrum Utilization.
New York: Joint Technical
Advisory Committee
(IEEE
and
EIA),
1964. CCIR XVth
Plenary Assembly, Geneva, 1982, Vol. VI.