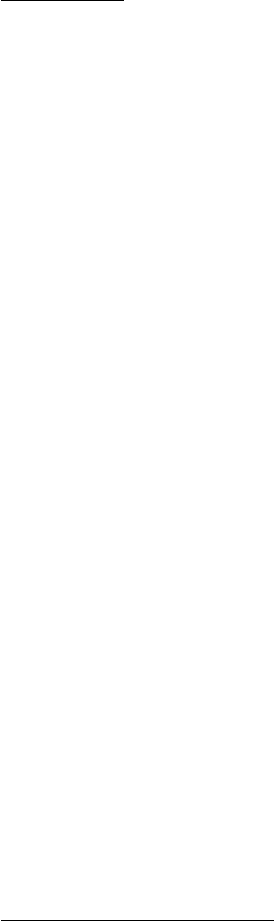
Each clan ranges widely in composition and fabric,
far more than common rock types. Additionally, there
are overlaps in most any rock property. Instead of tight
definitions, lengthy characterizations of each clan and
contrasts between them must suffice.
Lamproite Clan. Of the 24 known worldwide locales of
clustered lamproite bodies, altogether 100 km
3
, the
main locales are in southeastern Africa ( Jurassic),
Western Australia (Proterozoic and Miocene), and the
Leucite Hills of Wyoming (Quaternary). All lie in tec-
tonically stable plate interiors, although prior sub-
duction or rifting appears to have metasomatized the
thick continental lithosphere through which magmas
ascended. In Western Australia, lamproite occurs in
narrow pipes that flare at the surface into subcircular
craters. These are filled with a variety of volcaniclastic
deposits and are intruded by dikes and sills feeding
surface flows. The 2-Ma Leucite Hills consist of lava
flows and small cinder cones.
For comparison only (not as defining attributes),
chemical analyses in Table 13.11 indicate that, relative
to the orangeite and kimberlite clans, lamproites are rel-
atively rich in SiO
2
, TiO
2
, Ba (commonly 5000 ppm),
La (200), and Zr (500) and poor in CO
2
. When
compared to orangeites-kimberlites that contain abun-
dant carbonate minerals, fresh lamproites generally
have 0.5 wt. % CO
2
. They are ultrapotassic (molecu-
lar K
2
O/Na
2
O 3) and their peralkalinity is mani-
fested in Fe
3
-Ba-rich, Al-poor leucite and sanidine;
Ti-rich, Al-poor phlogopite; and Ti-K-rich richterite
amphibole. Also present are Mg-rich olivine (both phe-
nocrysts and groundmass in some), diopside, glass, and
accessory amounts of apatite, ilmenite, and exotic min-
erals, which include wadeite (K
2
ZrSi
3
O
9
), priderite (K,
Ba, Fe
3
, Ti oxide), perovskite (Ca, Na, Fe
2
, REEs,
Ti, Nb oxide), and several other minerals (Scott-Smith,
1996). Not all of these phases necessarily occur in a
single sample and some samples can be mineralogically
unique, unlike others in the clan. This attribute, to-
gether with dissimilarites between lamproites in differ-
ent locales and the fact that some lamproities contain
mantle-derived xenocrysts, imposes difficulties in accu-
rate characterization of the whole clan.
Orangeite and Kimberlite Clans. The orangeite and
kimberlite clans are hybrid rocks emplaced as explo-
sive breccia in carrot- and funnel-shaped diatremes
fed from intrusive, nonfragmental dike-sill complexes
(Figures 9.25 and 9.26). Rocks are exceptionally rich
(12 wt.%) in CO
2
H
2
O
, which are chiefly se-
questered in primary calcite and in secondary serpen-
tine that replaces olivine and orthopyroxene. The true
composition of the primitive magmas remains uncer-
tain because of differentiation and alteration overprints
and especially because of contamination by abundant
xenoliths and xenocrysts derived from both crust and
mantle. Xenoliths of crustal rock (shale, granite, and
others) and of mantle rock (eclogite and a wide vari-
ety of peridotitic rocks, commonly metasomatically al-
tered; see Section 11.2) are readily identified. On the
other hand, mantle-derived xenocrysts are difficult,
and commonly impossible, to distinguish from phe-
nocrysts that precipitated from the kimberlite melt at
different places in its excursion from depths of perhaps
200 km or more, where diamond is stable (Figure
11.5). The degree of euhedralism as a guide to discrim-
inating foreign versus cognate crystals may be mislead-
ing because deep euhedral precipitates might be re-
sorbed at shallower depths or physically abraded in
the explosive diatreme. Rocks are characteristically
inequigranular because the polygenetic megacrysts
(5 mm in diameter) are hosted in an aphanitic, never
glassy groundmass of calcite, secondary serpentine,
phlogopite, Mg-spinel, and apatite. Most megacrysts,
commonly about 25 modal %, are Mg-olivine, but in
archetypal kimberlite, lesser megacrysts include Mg-
rich ilmenite, phlogopite, enstatite, purple-red pyrope
(Mg-rich) garnet, and green Cr-rich clinopyroxene; the
latter two serve as colorful “indicator” minerals in the
regolith overlying weathered kimberlites and in down-
stream stream and glacial deposits.
Early work just after the turn of the 1900s revealed
two types of diamondiferous rocks in South Africa. In
subsequent decades, this distinction became confused,
but it was again clarified by Mitchell (1995). The two
clans are:
(1) Archetypal kimberlites (also called basaltic or
group I kimberlites)
(2) Orangeites (also called micaceous or group II kim-
berlites), which are mineralogically, isotopically,
and, with regard to origin, more similar to the lam-
proite clan than to archetypal kimberlites, although
orangeites contain more CO
2
and compatible Ni
and Cr but less Rb, Ba, Zr, and Ti than lamproites
The only systematic chemical contrast between the
kimberlites and orangeites lies in higher K
2
O/TiO
2
in
the latter. The most striking contrast is significantly
higher
87
Sr/
86
Sr and lower
143
Nd/
144
Nd in orangeites
than in archetypal kimberlites (Figure 13.41). Miner-
alogical contrasts among the lamproite, kimberlite, and
orangeite clans are set out in Table 13.12.
All known (200+) orangeite bodies lie in the
Archean Kaapvaal craton in South Africa (Figure
13.42), where they occur as early Cretaceous (125–
110 Ma) and early Jurassic (165–145 Ma) swarms of
dikes and diatremes. Worldwide, there are 5000
known bodies of archetypal kimberlites having an esti-
mated volume 5000 km
3
; most of these are in Pre-
cambrian cratons. They typically occur in clusters
40 km across of a few dozen intrusions (Nixon,
1995). In southern Africa, kimberlite emplacement has
occurred episodically several times since 1600 Ma.
Magmatic Petrotectonic Associations
399