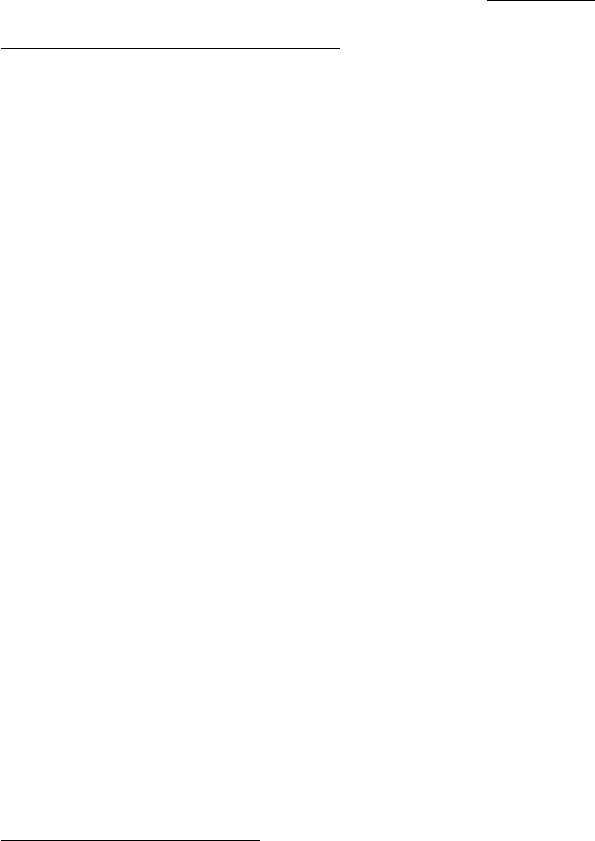
(aphanitic) and foliated mylonite. In a metamorphic
rock classification based solely on fabric, this rock
qualifies as a slate, despite its granitoid protolith! The
name “slate” carries no connotation as to origin or
protolith, although to most readers slates are derived
from shales.
Transformation of Graywacke into Schist. A thick se-
quence of rocks in the South Island of New Zealand
(see Figure 18.30) shows progressive metamorphism
and development of tectonite fabric from an initially
isotropic protolith of feldspathic and lithic sandstones,
sometimes called graywackes (Figure 14.17). The
changes in these rocks are a result not simply of deep
burial at increasing P and T but also of increasing
degree of recrystallization under nonhydrostatic stress.
Widespread folds attest to the deformation in these
tectonites. Although the feldspar and quartz grains in
the final end result—a schist—have 120° triple junc-
tions resembling granoblastic texture, the aligned platy
minerals and slightly flattened felsic minerals define an
anisotropic tectonite fabric. Significantly, in the more
recrystallized, coarser rocks, the foliation expressed
by the aligned platy minerals is augmented by parallel
layers of contrasting composition that are another ex-
pression of metamorphic differentiation. Like growth
of porphyroblasts, mineral components moved dif-
ferentially during recrystallization but rather than to
isolated crystal nuclei they somehow segregated into
planar layers. This ordering seems to defy the law of
spontaneously increasing entropy. Whatever its origin,
metamorphic differentiation can create compositional
layers where none previously existed. Hence, com-
positional layers in a metasedimentary rock do not
necessarily represent relict bedding. Careful attention
must be paid to the degree of recrystallization, grain
size, and possible preservation of a relict fabric, as well
as field relations, to accurately interpret the origin of
compositional layering.
Slaty and Crenulation Cleavage. Slates possess a slaty
cleavage defined by aligned aphanitic platy phyllosilic-
ates (micas and chlorite) and commonly minute flakes
of graphite. Thin, quartz-rich layers and less continu-
ous lenses may also occur and these can be locally, but
not necessarily, subparallel to the slaty cleavage. Subtle
gradational changes in grain size within the quartz-rich
layers, together with the very fine overall grain size of
the rock, suggest little recrystallization has occurred.
Hence, it is reasonable to interpret the compositional-
textural layering as relict sedimentary interbeds of
siltstone and sandstone in shale. Low-grade meta-
morphism created bedding-parallel flakes of micas
and chlorites that nucleated and grew in the pro-
tolith, mimicking the orientation of the original flat
clay particles. In a later deformation event this slaty
cleavage can be crenulated so that limbs of the over-
printing crenulations define a second, oblique foliation
—a crenulation cleavage (Figure 14.18). This is
another example of polymetamorphism.
Transposition. Existing compositional layers or other
types of foliation in a metamorphic body can be
sheared or smeared out into a new and different orien-
tation during ductile deformation and recrystallization.
An example of this transposition related to ductile
folding of layers is displayed in Figure 14.19b. Note the
flattening perpendicular to the new metamorphic foli-
ation and corresponding extension parallel to it. Trans-
position occurs on a wide range of scales, from thin
section to mountain range. Figure 14.20 shows that
pillows of lava can also be transposed into thin lenses;
with continued ductile deformation under nonhydro-
static stress these could become more flattened. Once
again, thin lenses and even layers can be created in a
tectonite where none previously existed.
14.1.4 Crystalloblastic Series
In the tectonite in Figure 14.17d, grain boundaries
appear to have equilibrium shapes resembling those in
granoblastic texture, except for two attributes. First,
inequant platy micas have a strong preferred shape
orientation that precludes use of the term granoblastic
as conventionally defined. Second, careful examination
reveals that micas are relatively euhedral in contact
with anhedral grains of quartz and oligoclase but in
contact with euhedral garnet are decidedly anhedral.
Hence, these four minerals manifest a hierarchy of
development of characteristic crystal forms during
solid-state growth. The mutual competition for com-
mon space during such growth is distinctly different
from crystallization in melts where each growing grain
—at least near the liquidus—is not affected in its shape
by growing neighbors. This hierarchy of euhedralism in
metamorphic rocks was first recognized by F. Becke in
1909 and called a crystalloblastic series. It can be seen
in all sorts of metamorphic fabrics—anisotropic as well
as isotropic. In the following list of metamorphic rock-
forming minerals, denser ones having tighter packing
of ions tend to be more euhedral as they compete for
space during recrystallization, as follows:
MOST EUHEDRAL
Titanite, rutile, pyrite
Garnet, sillimanite, staurolite, tourmaline
Epidote, magnetite, ilmenite
Andalusite, pyroxene, amphibole
Micas, chlorite, dolomite, kyanite
Calcite, vesuvianite, scapolite
Feldspar, quartz, cordierite
LEAST EUHEDRAL
Metamorphic Rocks and Metamorphism: An Overview
421