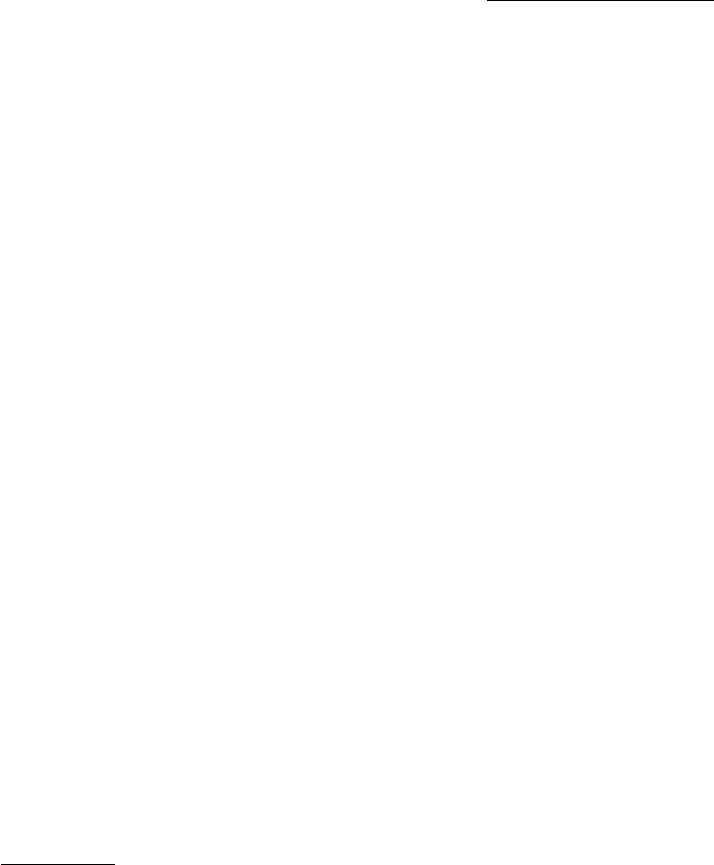
facies metamorphism. Although much remains to be
learned, his conception of the zeolite and prehnite–
pumpellyite facies has withstood the test of innumer-
able subsequent investigations of arc rocks all over the
world.
Investigation of these rocks presents several chal-
lenges. Relict fabrics and minerals of the protoliths are
preserved, seriously hampering the interpretation of
overprinting metamorphic mineral assemblages. Newly
equilibrated low-T minerals are typically very fine
grained and commonly occur as fringes on pre-existing
primary mineral grains. Whether new minerals con-
stitute an equilibrium assemblage can always be ques-
tioned and whether they develop at all depends on the
availability of a penetrating fluid; this is particularly
acute in the case of mafic magmatic rock protoliths that
are initially volatile free. Whereas more permeable sedi-
mentary and tuffaceous rocks, especially where glassy
(Figure 14.2), can experience more pervasive recrystal-
lization resulting from invaded fluids, interlayered
basaltic and andesitic rocks are less susceptible to
equilibration under changing metamorphic conditions.
This is particularly the case where the rock mass re-
mains undeformed without avenues for invasion of
fluids. The composition of the penetrating fluid can
also be crucial; Thompson (1971) showed that only
minute concentrations of CO
2
(X 0.0075) in a
aqueous fluid are sufficient to stabilize calcite kaoli-
nite quartz in lieu of laumontite. Thus, in many
metamorphic terranes possibly flooded by fluids
sufficiently rich in CO
2
, zeolite-facies and possibly
prehnite–pumpellyite-facies assemblages are absent
and the onset of metamorphism is marked by greenschist-
facies assemblages.
Understanding the paragenesis of the zeolite and
prehnite–pumpellyite facies formed under the lowest
P–T conditions of metamorphism provides a perspect-
ive on transitions into the greenschist and blueschist
facies formed at higher T and P (Figure 14.33) that
occur in nearby geologic settings (Figure 14.24). How-
ever, a lack of well calibrated mineral thermobarome-
ters hinders the interpretation of the lower T rocks and
creates inaccuracies in P–T paths. Equilibration, partial
or complete, at temperatures less than closure tem-
peratures also hinders determination of chronologic
aspects of metamorphism.
Zeolite Facies. Although Eskola recognized the low-T
existence of zeolitic mineral assemblages as early as
the 1930s, it was not until Coombs et al. (1959) docu-
mented regular mineralogical zones in regionally ex-
tensive zeolite-bearing rocks in southern New Zealand
(Figure 18.30) that the zeolite facies was proposed.
Here, during the Triassic, locally as much as 9 km of
volcanogenic clastic material accumulated in a subsid-
ing trough adjacent to a volcanic arc. As the thickening
CO
2
pile was subjected to burial metamorphism, the reac-
tive, commonly glassy volcanic detritus recrystallized
into very fine-grained zeolites (Figure 14.3) and other
low-T phases, including albite and epidote. The zeo-
lites stilbite and mordenite developed at shallower
depths and lower temperatures, whereas heulandite
and then laumontite and wairakite formed at higher
temperatures. Miyashiro (1994) considers any equili-
brated assemblage of zeolite quartz to belong to the
zeolite facies.
Prehnite–Pumpellyite Facies. In the deeper part of the
thick Mesozoic pile of arc-derived clastic rocks in
southernmost New Zealand, prehnite and pumpellyite
appear together with laumontite and other zeolites. But
in similar volcanogenic rocks elsewhere in the South
Island of New Zealand, in Japan, and in other areas of
the world, zeolites and quartz are not stable with
prehnite pumpellyite. (Pumpellyite (Table 14.1 and
Appendix A) is a bluish-green epidote-like mineral
with a composition roughly equivalent to epidote
actinolite water.) However, prehnite and pumpel-
lyite occur with epidote chlorite and other phases
typical of the lower greenschist facies, including acti-
nolite, albite, stilpnomelane, white mica, titanite, and
calcite, or with lawsonite albite and other phases
typical of the blueschist facies. Coombs (1961) desig-
nated widespread assemblages of minerals that are
transitional between the zeolite and the greenschist or
blueschist facies the prehnite–pumpellyite facies. Mafic
rocks of this facies typically have a distinct bluish-green
color because of abundant pumpellyite. Schmidt
and Robinson (1997) have made a detailed analysis
of the transition from smectites to chlorites in meta-
basites equilibrated under zeolite- to greenschist-facies
conditions.
Prehnite is not stable in the highest grade assem-
blage of the prehnite–pumpellyite facies that has coex-
isting actinolite pumpellyite chlorite epidote.
This four-phase assemblage would seem to be imposs-
ible in the three-component ACF system of Figure
18.31, but additional components such as Fe
3
and Ti
may account for its stabilization.
The stability field of prehnite pumpellyite ranges
from less than 200°C to about 300°C and from about
4 kbars to 1 kbar, where the two minerals are stabil-
ized in seafloor metamorphic rocks. With increasing
T, these two phases react with chlorite and quartz to
produce epidote actinolite, marking the transition
into the greenschist facies. In rocks poor in chlorite
or quartz, pumpellyite or prehnite can persist stably
into the greenschist-facies regime to temperatures
approaching 400°C. Interpretation of mafic mineral
assemblages that contain pumpellyite, prehnite, epi-
dote, and actinolite is fraught with problems, probably
in part kinetic (Beiersdorfer and Day, 1995).
598 Igneous and Metamorphic Petrology