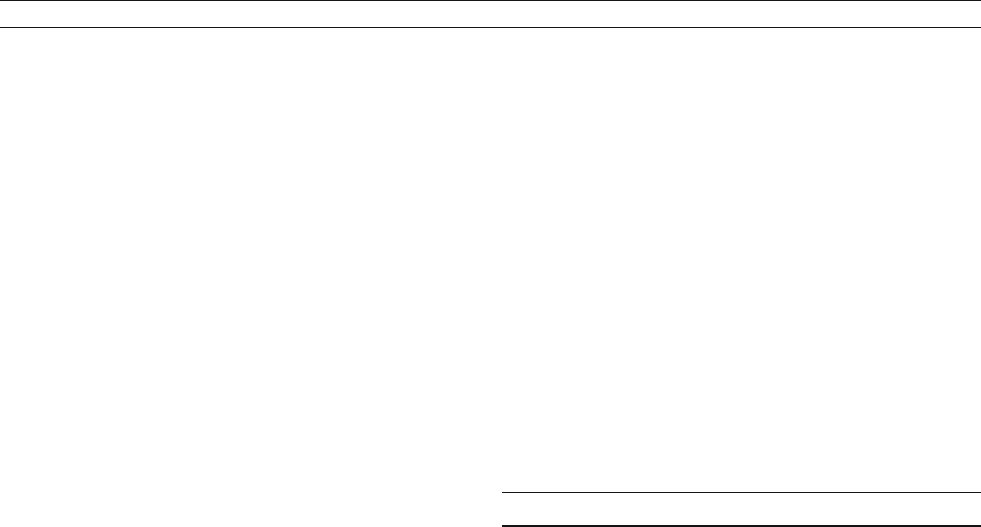
Butcher, S.S., Charlson, R.J., Orians, G.H., and Wolfe, G.V. (eds.) 1992.
Global Biogeochemical Cycles. London/ San Diego,CA: Academic
Press, 379pp.
Christensen, B.A., and Giraudeau J. (eds.) 2002. Neogene and quaternary
evolution of the Benguela coastal upwelling system. Mar. Geol., 180
(Special Issue), 1–276.
CLIMAP Project Members, 1976. The surface of the ice-age Earth.
Science, 191, 1131–1137.
Daly, R.A., 1934. The Changing World of the Ice Age. New Haven: Yale
University Press, 271pp.
Dickens, G.R., O’Neil, R.R., Rea, D.K., and Owen, R.M., 1995.
Dissociation of oceanic methane hydrate as a cause of the carbon
isotope excursion at the end of the Paleocene. Paleoceanography, 10,
965–971.
Emiliani, C., 1955. Pleistocene temperatures. J. Geol., 63, 538–578.
Fairbanks, R.G., 1989. A 17,000-year glacio-eustatic sea level record:
Influence of glacial melting rates on the Younger Dryas event and
deep-ocean circulation. Nature, 342, 637–642.
Falkowski, P.G., and Woodhead, A.D. (eds.) 1992. Primary Productivity
and Biogeochemical Cycles in the Sea. New York: Plenum Press,
550pp.
Fischer, G., and Wefer, G. (eds.) 1999. Use of Proxies in Paleoceanogra-
phy. Berlin: Springer, 735pp.
Flohn, H., 1985. Das Problem der Klimaänderungen in Vergangenheit und
Zukunft. Darmstadt: Wissenschaftliche Buchgesellschaft, 228pp.
Funnell, B.M., and Riedel, W.R. (eds.) 1971. The Micropalaeontology of
Oceans. Cambridge, UK: Cambridge University Press, 828pp.
Haq, B.U., 1981. Paleogene paleoceanography: Early Cenozoic oceans
revisited. Oceanologica Acta, 4 (Supplement), 71–82.
Hay, W.W., 1988. Paleoceanography: A review for the GSA centennial.
Geol. Soc. Am. Bull., 100, 1934–1956.
Hays, J.D., Imbrie, J., and Shackleton, N.J., 1976. Variations in the Earth’s
orbit: Pacemaker of the ice ages. Science, 194, 1121–1132.
Kennett, J.P., 1982. Marine Geology. Englewood Cliffs, NJ: Prentice-Hall,
813pp.
Kennett, J.P. (ed.) 1985. The Miocene Ocean: Paleoceanography and bio-
geography. Geol. Soc. Am. Memoir, 163
,1–337.
Luterbacher, H.P., and Premoli-Silva, I., 1964. Biostratigrafia del limite
Cretaceo-Terziario. Riv. Ital. Paleontol., 70,67–128.
Milankovitch, M., 1930. Mathematische Klimalehre und astronomische
Theorie der Klimaschwankungen. Handbuch der Klimatologie, Bd 1,
Teil A, Berlin, Bornträger, 176pp.
Miller, K.G., Fairbanks, R.G., and Mountain, G.S., 1987. Tertiary oxygen
isotope synthesis, sealevel history, and continental margin erosion.
Paleoceanography, 2,1–19.
Olausson, E., 1965. Evidence of climatic changes in North Atlantic deep-
sea cores, with remarks on isotopic paleotemperature analysis. Prog.
Oceanogr., 3, 221–252.
Parker, F.L., 1962. Planktonic foraminiferal species in Pacific sediments.
Micropaleontology, 8(2), 219–254.
Prothero, D.R., and Berggren, W.A. (eds.) 1992. Eocene-Oligocene Climatic
and Biotic Evolution. Princeton, NJ: Princeton University Press, 566pp.
Purdy, E.G., 1974. Reef configurations: Cause and effect. In Laporte, L.F.
(ed.), Reefs in Time and Space. Tulsa, OK: Society of Economic
Paleontologists and Mineralogists. SEPM Special Publication 18,
pp. 9–76.
Reading, H.G. (ed.) 1986. Sedimentary Environments and Facies, 2nd edn.
Oxford: Blackwell Scientific, 615pp.
Seibold, E., and Berger, W.H., 1993. The Sea Floor. 3rd edn. Berlin:
Springer, 356pp.
Shackleton, N., and Crowhurst, S., 1994. Details that make the difference.
Oceanus, 36(4), 45–48.
Summerhayes, C.P., Prell, W.L., and Emeis, K.C. (eds.) 1992. Upwelling
Systems: Evolution since the Early Miocene. Piccadilly: Geological
Society of London. Geological Society of London Special Publication
64, 519pp.
Thierstein, H.R., and Okada, H., 1979. The Cretaceous/Tertiary boundary
event in the North Atlantic. Initial Rep. Deep Sea Drill. Proj., 43, 601–616.
van Andel, Tj.H., Thiede, J., Sclater, J.G., and Hay, W.W., 1977. Deposi-
tional history of the South Atlantic Ocean during the last 125 million
years. J. Geol., 85, 651–698.
Vincent, E., and Berger, W.H., 1981. Planktonic foraminifera and their use
in paleoceanography. In Emiliani, C. (ed.),“The Sea, vol. 7, the Oceanic
Lithosphere.” New York: Wiley-Interscience, pp. 1025–1119.
Wefer, G., and Berger, W.H., 1991. Isotope paleontology: Growth and com-
position of extant calcareous species. Mar. Geol.,
100, 207–248.
Winter, A., and Siesser, W.G., 1994. Coccolithophores. Cambridge, UK:
Cambridge University Press, 242pp.
Cross-references
Astronomical Theory of Climate Change
Carbonate Compensation Depth
CLIMAP
Coccoliths
Coral and Coral Reefs
Cretaceous/ Tertiary (K-T) Boundary Impact, Climate Effects
Deep Sea Drilling Project (DSDP)
Diatoms
Foraminifera
Ocean Anoxic Events
Ocean Drilling Program (ODP)
Paleocene-Eocene Thermal Maximum
Quaternary Climate Transitions and Cycles
Radiolaria
MARINE CARBON GEOCHEMISTRY
Introduction
The ocean contains approximately 50 times as much dissolved
CO
2
as is contained in the atmosphere, and the carbon cycle in
the ocean controls the pCO
2
of the atmosphere on time scales
of thousands of years. The atmospheric CO
2
concentration
today is rising because of human activities, such as fossil fuel
combustion and deforestation. The CO
2
rise is faster than the
century-scale ocean/atmosphere equilibration time, but the
ocean will ultimately absorb most of the CO
2
that is released.
In the past, changes in the carbon cycle of the ocean drove
changes in atmospheric pCO
2
synchronously with the glacial/
interglacial climate cycles. The orbital forcing which paces
the glaciations (called Milankovitch cycles, see Astronomical
Theory of Climate Change) is asynchronous across the equator;
extra warmth in the north is balanced by decreased heating in
the south. However, because of the rapid mixing of the atmo-
sphere, the glacial CO
2
cycles tend to warm or cool the entire
planet synchronously, and are largely responsible for the global
synchrony of the glaciations. The carbon cycle of the ocean is
therefore a primary determiner of the climate of the Earth, in
the past and in the future.
The carbon cycle in the ocean begins with gas fluxes across
the sea surface into the atmosphere. These depend on wind
speed, temperature, and the chemistry of the water at the sea
surface. Seawater chemistry of CO
2
is complicated by the pre-
sence of the carbonate pH buffer system pH. Only a tiny frac-
tion of the dissolved carbon is present as the uncharged
dissolved gas that can interact with the atmosphere. Because
of this chemistry, a slight change in the pH of seawater can
result in an enormous change in the concentration of dissolved
CO
2
, and in the equilibrium pressure of CO
2
in the atmosphere.
The solubility of dissolved CO
2
depends on temperature and to
a lesser extent salinity, so that a warming parcel of seawater
will tend to push more CO
2
into the atmosphere. The CO
2
con-
centration of surface ocean waters is also affected by the
growth and sinking of phytoplankton in the surface ocean.
The carbon cycle of the ocean is therefore determined by a
MARINE CARBON GEOCHEMISTRY 533