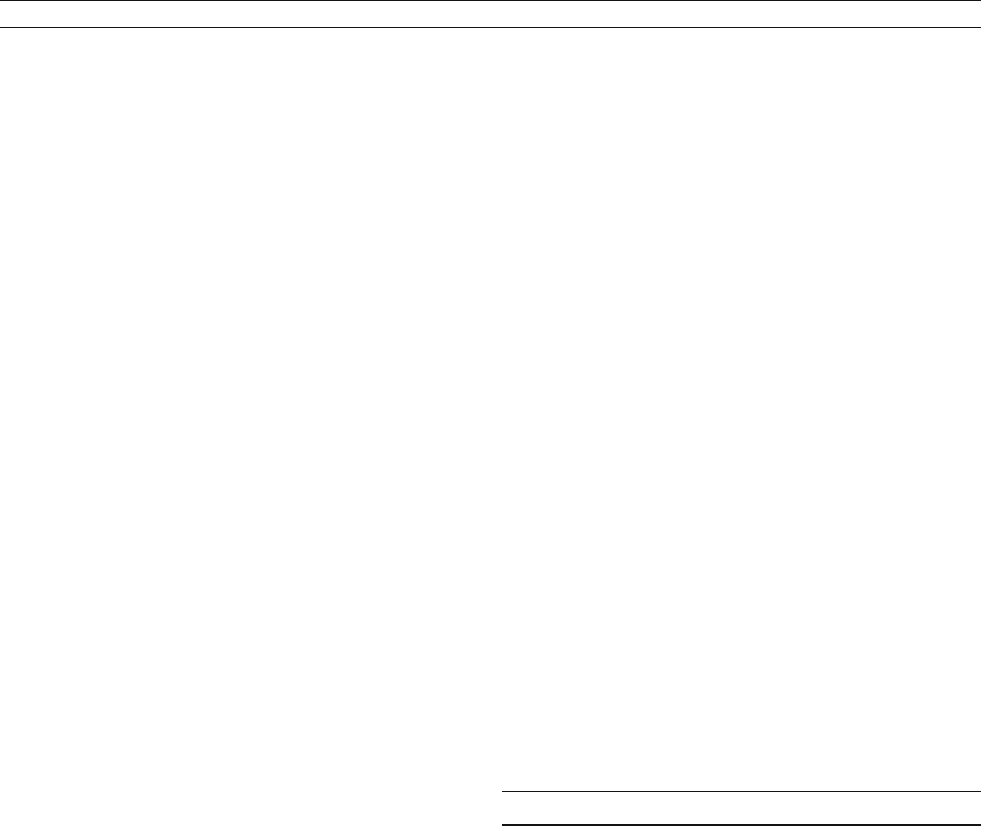
Byrne, S., and Ingersoll, A.P., 2003. A sublimation model for Martian
south polar ice features. Science, 299, 1051–1053.
Carr, M.H., 2001. Mars global surveyor observations of fretted terrain.
J. Geophys. Res., 106, 23571–23595.
Christensen, P.R., 2003. Formation of recent Martian gullies through
melting of extensive water-rich snow deposits. Nature, 422,45–48,
doi:10.1038/ nature01436.
Christensen, P.R., and Ruff, S.W., 2004. The formation of the hematite-
bearing unit in Meridiani Planum: Evidence for deposition in standing
water. J. Geophys. Res., 109, E08003, doi:10.1029/ 2003JE002233.
Christensen, P.R., Bandfield, J.L., Hamilton, V.E., Ruff, S.W., Kieffer, H.
H., Titus, T., Malin, M.C., Morris, R.V., Lane, M.D., Clark, R.N.,
Jakosky, B.M., Mellon, M.T., Pearl, J.C., Conrath, B.J., Smith, M.D.,
Clancy, R.T., Kuzmin, R.O., Roush, T., Mehall, G.L., Gorelick, N.,
Bender, K., Murray, K., Dason, S., Greene, E., Silverman, S.H., and
Greenfield, M., 2001. The Mars Global Surveyor Thermal Emission
Spectrometer experiment: Investigation description and surface science
results. J. Geophys. Res., 106, 23823–23871.
Christe nsen, P.R., McSween, H.Y. Jr., Bandfield, J.L., Ruff, S.W.,
Rogers, A.D., Hamilton, V.E., Gorelick, N., Wyatt, M.B., Jakosky,
B.M., Kieffer, H.H., Malin, M.C., and Moersch, J.E., 2005.
Evid ence for igneous diversity and magmatic evolution on Mars
from infrared spectral observations, Nature, 436, doi:10.1038 /
nature03639.
Clow, G.D., 1987. Generation of liquid water on Mars through the melting
of a dusty snowpack. Icarus, 72,95–127.
Feldman, W.C., Boynton, W.V., Tokar, R.L., Prettyman, T.H., Gasnault, O.,
Squyres, S.W., Elphic, R.C., Lawrence, D.J., Lawson, S.L., Maurice, S.,
McKinney, G.W., Moore, K.R., and Reedy, R.C., 2002. Global distri-
bution of neutrons from Mars: Results from Mars Odyssey. Science,
297,75–78.
Gendrin, A., Mangold, N., Bibring, J.-P., Langevin, Y., Gondet, B.,
Poulet, F., Bonello, G., Quantin, C., Mustard, J., Arvidson, R., and
LeMouelic, S., 2005. Sulfates in Martian layered terrains: The
OMEGA/ Mars Express view. Science, 307, 1587–1590.
Glotch, T.D., and Christensen, P.R., 2005. Geologic and mineralogic
mapping of Aram Chaos: Evidence for a water-rich history. J. Geophys.
Res., 110, E09006, doi:10.1029/ 2004JE2389.
Grotzinger, J., Bell, J.F. III., Calvin, W., Clark, B.C., Fike, D.,
Golombek, M., Greeley, R., Herkenhoff, K.E., Jolliff, B., Knoll, A.H.,
Malin, M., McLennan, S.M., Parker, T., Soderblom, L., Sohl-Dickstein,
J.N., Squyres, S.W., Tosca, N.J., and Watters, W., 2005. Stratigraphy,
sedimentology and depositional environment of the Burns Formation,
Meridiani Planum, Mars. Earth Planet. Sci. Lett., 240,14–75.
Gulick, V.C., and Baker, V.R., 1989. Fluvial valleys and Martian palaeo-
climates. Nature, 341, 514–516.
Hamilton, V.E., and Christensen, P.R., 2005. Evidence for extensive
olivine-rich bedrock in Nili Fossae, Mars. Geology, 33, 433–436.
Heldmann, J.L., and Mellon, M.T., 2004. Observations of Martian gullies
and constraints on potential formation mechanisms. Icarus, 168,
285–304.
Hoefen, T., Clark, R.N., Bandfield, J.L., Smith, M.D., Pearl, J.C., and
Christensen, P.R., 2003. Discovery of olivine in the Nili Fossae region
of Mars. Science, 302, 627–630.
Howard, A.D., Moore, J.M., and Irwin, R.P. III., 2005. An intense terminal
epoch of widespread fluvial activity on early Mars: 1. Valley network
incision and associated deposits. J. Geophys. Res., 110, E12S14,
doi:10.1029/ 2005JE002459.
Jakosky, B.M., and Carr, M.A., 1985. Possible precipitation of ice at low
latitudes of Mars during periods of high obliquity. Nature, 315,
559–561.
Kieffer, H.H., 1979. Mars south polar spring and summer temperatures:
A residual CO
2
frost. J. Geophys. Res., 84, 8263–8289.
Langevin, Y., Poulet, F., Gendrin, A., Gondet, B., Berthé, M., Soufflot, A.,
Drossart, P., Combes, M., Bellucci, G., Moroz, V., Mangold, N.,
Schmitt, B., and Omega-Team, 2004. Perennial water ice identified in
the south polar cap of Mars. Nature, 428, 627–630.
Lee, P., Cockell, C.S., Marinova, M.M., McKay, C.P., and Rice, J.W., 2001.
Snow and ice melt flow features in Devon Island, Nunavut, Arctic
Canada as possible analogs for recent slope flow features on Mars, in
Proceedings of the 32nd Lunar and Planetary Science Conference,
abstract 1809, March 12–16, Houston, Texas [CD-ROM].
Malin, M.C., and Edgett, K.S., 2000. Evidence for recent ground water
seepage and surface runoff on Mars. Science, 288, 2330–2335.
Malin, M.C., and Edgett, K.S., 2003. Evidence for persistent flow and
aqueous sedimentation on early Mars. Science, 302, 1931–1934.
McSween, H.Y. Jr., et al., 2004. Basaltic rocks analyzed by the Spirit rover
in Gusev Crater. Science, 305, 842–845.
Mellon, M.T., and Phillips, R.J., 2001. Recent gullies on Mars and the
source of liquid water. J. Geophys. Res., 106, 23165–23180.
Mustard, J.F., Cooper, C.D., and Rifkin, M.K., 2001. Evidence for recent
climate change on Mars from the identification of youthful near-surface
ground ice. Nature, 412,411–414.
Mustard, J.F., Poulet, F., Gendrin, A., Bibring, J.-P., Langevin, Y., Gondet, B.,
Mangold, N., Bellucci, G., and Altieri, F., 2005. Olivine and pyroxene
diversity in the crust of Mars. Science, 307,1594–1597.
Pollack, J.B., and Toon, O.B., 1982. Quasi-periodic climate changes on
Mars: A review. Icarus, 50, 259–287.
Segura, T.L., Toon, O.B., Colaprete, A., and Zahnle, K., 2002. Environ-
mental effects of large impacts on Mars. Science, 298, 1977–1980.
Soderblom, L.A., 1992. The composition and mineralogy of the Martian
surface from spectroscopic observations: 0.3 mmto50mm. In
Kieffer, H.H., Jakosky, B.M., Snyder, C.W., and Matthews, M.S.
(eds.), Mars. Tucson, AZ: University of Arizona Press, pp. 557–593.
Squyres, S.W., Grotzinger, J.P., Bell, J.F., Christensen, P.R., Clark, B.C.,
Crisp, J.A., Farrand, W.H., Herkenhoff, K.E., Klingelhöfer, G.,
Knoll, A.H., McLennan, S.M., McSween, H.Y., Morris, R.V.,
Rieder, R., and Soderblom, L.A., 2004. In-situ evidence for an ancient
aqueous environment on Mars. Science, 306, 1709–1714.
Titus, T.N., Kieffer, H.H., and Christensen, P.R., 2003. Exposed water
ice discovered near the south pole of Mars. Science, 299, 1048–1051.
Ward, W.R., 1974. Climatic variations on Mars. I. Astronomical theory
of insolation. J. Geophys. Res., 79, 3375–3386.
Wyatt, M.B., McSween, H.Y. Jr., Tanaka, K.L., and Head, J.W. III., 2004.
Global geologic context for rock types and surface alteration on Mars.
Geology, 32, 645–648, doi:10.1130/G20527.1.
Cross-references
Astronomical Theory of Climate Change
Atmospheric Evolution, Mars
Mineral Indicators of Past Climates
Obliquity
Sedimentary Indicators of Climate Change
MASS EXTINCTION: ROLE OF CLIMATE
Introduction
Mass extinction is a defining feature of life’shistory.Over
short periods of geologic time, geographically widespread and
ecologically diverse groups of organisms disappear from the fossil
record. The five largest extinctions of the Phanerozoic occurred
during the Late Ordovician, Late Devonian, and at the Permo-
Triassic, Triassic-Jurassic, and Cretaceous-Tertiary boundaries,
when over 40% of genera and 60% of species became extinct.
Some mass extinctions completely restructured many components
of the world’s marine ecosystems, while others caused major loss
of species but little ecological restructuring.
Major changes in the Earth’s climate system have been
proposed to explain mass extinctions and their associated geo-
chemical anomalies. Current debate focuses on the nature of
climatic change during mass extinction and whether it results from
extra-terrestrial perturbations of the Earth system or from internal
processes. Additional debate centers on whether changes in the
physical climate system or chemistry of the atmosphereand oceans
are most important. The search for the environmental cause(s) of
mass extinction is a dynamic field with a voluminous literature.
Outlined below are climatic mechanisms proposed for the five lar-
gest Phanerozoic extinctions and a few important references.
MASS EXTINCTION: ROLE OF CLIMATE 547