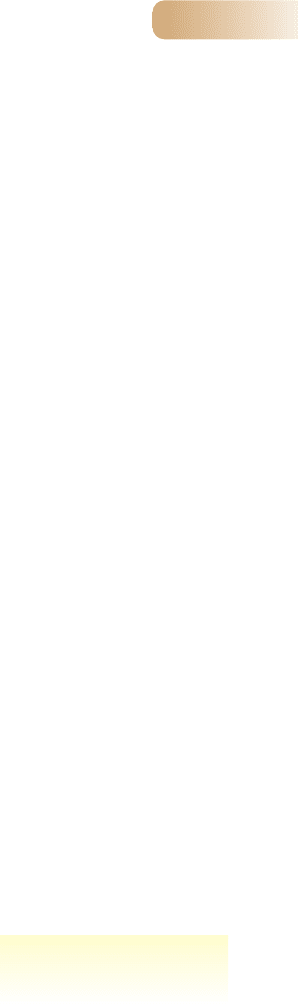
any of the various terrestrial biomes, but this is largely due to their close relation-
ship with major terrestrial climates. In contrast, the open oceans form a continuum
in which there is flow of water and dissolved chemicals across the globe. We have
seen how variation in the intensity of solar radiation from place to place and
between the seasons has dramatic effects on the temperature and water relations of
terrestrial environments. But this is not the case in the oceans. The high thermal
capacity of water makes the oceans slow to heat and slow to cool. One effect is that
the temperature of the water at one point on the globe is a better reflection of where
the water has come from (along ocean currents) than of the local climate.
The world’s large lakes can be distinguished and classified according to their
physical conditions. For example, large lakes in lowland equatorial regions gener-
ally experience permanent stratification (distinct layers of water at particular
temperatures), whereas seasonal patterns of stratification (in summer) and mixing
(in fall) are the rule in temperate regions. Within the polar circles, permanent ice
cover with no mixing is characteristic of large lakes. However, local geological
conditions and basin size and shape have strong influences on conditions and
resources in lakes, particularly in terms of water chemistry, a key determinant of
lake flora and fauna. Consequently, a broad geographic classification of lake com-
munities has only limited merit. In the case also of streams, rivers, estuaries and
the open ocean, we will see that local conditions and resources are paramount in
determining community patterns (see Section 4.5).
4.3 Temporal patterns in conditions
and resources
The composition of communities can change over time scales ranging from hours
to millennia, as conditions and resources themselves change. For example, the
microbial community that colonizes and decomposes a dead mouse or fragment
of a leaf may change from hour to hour. At the other extreme, we can trace
patterns in community composition over tens of thousands of years. Thus, changes
in climate during the Pleistocene ice ages bear much of the responsibility for
present patterns of distribution of plants and animals. In the 20,000 years since
the peak of the last glaciation, global temperatures have risen by about 8°C. Many
tree species continue, even today, to migrate northward, following the retreat of
the glaciers (Figure 4.7).
At intermediate temporal scales, predictable sequences of plant species may
occur over periods ranging from years to centuries. For example, the successional
sequence that occurs on cooled volcanic lava takes several centuries to run its
course. This has been documented by comparing the plants living on lava flows
from eruptions that occurred at different times on Miyake-jima Island, Japan
(Figure 4.8). In the earliest stage of succession, conditions are harsh and soil
is sparse and lacking in nitrogen-containing ions, an essential plant resource.
Alders are first to colonize because they can fix atmospheric nitrogen into usable
form. As nitrogen availability in the soil increases, many species of fern, herb,
liana and tree enter the succession. After a century or two, late-successional trees
(Machilus then Castanopsis) shade out many of the earlier arrivals. Succession – the
predictable sequence of colonization and extinction after a disturbance – depends
partly on changing conditions and resources, and partly on the differential com-
petitive abilities of the plants themselves, a topic we return to in Chapter 9.
Chapter 4 Conditions, resources and the world’s communities
117
plant succession – the species
sequence on volcanic lava flows
9781405156585_4_004.qxd 11/5/07 14:47 Page 117