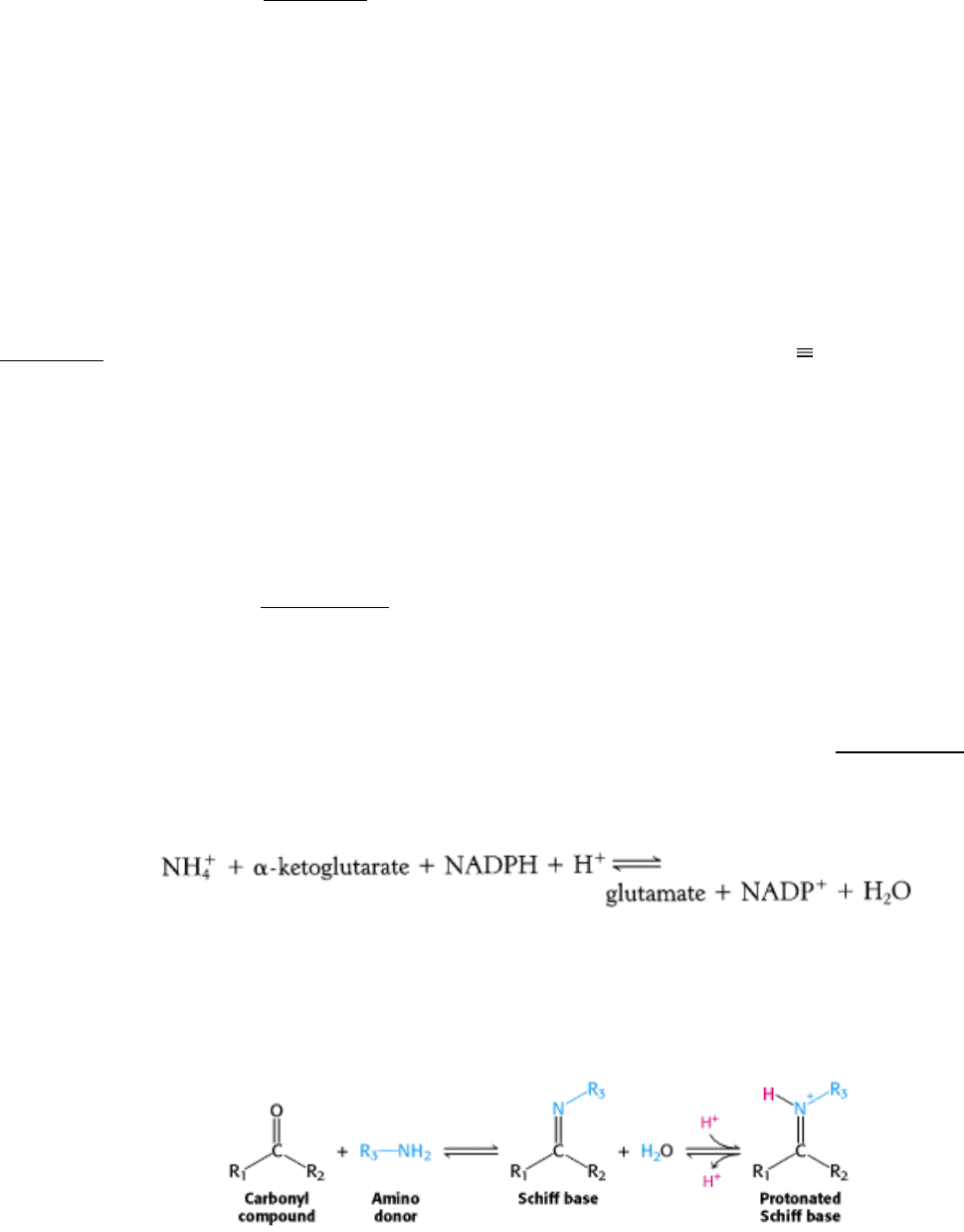
Thus, we see another example of how this domain has been recruited in evolution because of its ability to couple
nucleoside triphosphate hydrolysis to conformational changes.
The nitrogenase component is an α
2
β
2
tetramer (240 kd), in which the α and β subunits are homologous to each other
and structurally quite similar (Figure 24.4). Electrons enter at the P clusters, which are located at the α-β interface.
These clusters are each composed of eight iron atoms and seven sulfide ions. In the reduced form, each cluster takes the
form of two 4Fe-3S partial cubes linked by a central sulfide ion. Each cluster is linked to the protein through six
cysteinate residues. Electrons flow from the P cluster to the FeMo cofactor, a very unusual redox center. Because
molybdenum is present in this cluster, the nitrogenase component is also called the molybdenum-iron protein (MoFe
protein). The FeMo cofactor consists of two M-3Fe-3S clusters, in which molybdenum occupies the M site in one cluster
and iron occupies it in the other. The two clusters are joined by three sulfide ions. The FeMo cofactor is also coordinated
to a homocitrate moiety and to the α subunit through one histidine residue and one cysteinate residue. This cofactor is
distinct from the molybdenum-containing cofactor found in sulfite oxidase and apparently all other molybdenum-
containing enzymes except nitrogenase.
The FeMo cofactor is the site of nitrogen fixation. Note that each of the six central iron atoms is linked to only three
atoms, leaving open a binding opportunity for N
2.
It seems likely that N
2
binds in the central cavity of this cofactor
(Figure 24.5). The formation of multiple Fe-N interactions in this complex weakens the N N bond and thereby lowers
the activation barrier for reduction.
24.1.2. Ammonium Ion Is Assimilated into an Amino Acid Through Glutamate and
Glutamine
The next step in the assimilation of nitrogen into biomolecules is the entry of NH
4
+
into amino acids. Glutamate and
glutamine play pivotal roles in this regard. The α-amino group of most amino acids comes from the α-amino group of
glutamate by transamination (Section 23.3.1). Glutamine, the other major nitrogen donor, contributes its side-chain
nitrogen atom in the biosynthesis of a wide range of important compounds, including the amino acids tryptophan and
histidine.
Glutamate is synthesized from NH
4
+
and α-ketoglutarate, a citric acid cycle intermediate, by the action of glutamate
dehydrogenase. We have already encountered this enzyme in the degradation of amino acids (Section 23.3.1). Recall that
NAD
+
is the oxidant in catabolism, whereas NADPH is the reductant in biosyntheses. Glutamate dehydrogenase is
unusual in that it does not discriminate between NADH and NADPH, at least in some species.
The reaction proceeds in two steps. First, a Schiff base forms between ammonia and α-ketoglutarate.The formation of a
Schiff base between an amine and a carbonyl compound is a key reaction that takes place at many stages of amino acid
biosynthesis and degradation.
Schiff bases can be easily protonated. With glutamate dehydrogenase, the protonated Schiff base is reduced by the
transfer of a hydride ion from NADPH to form glutamate.