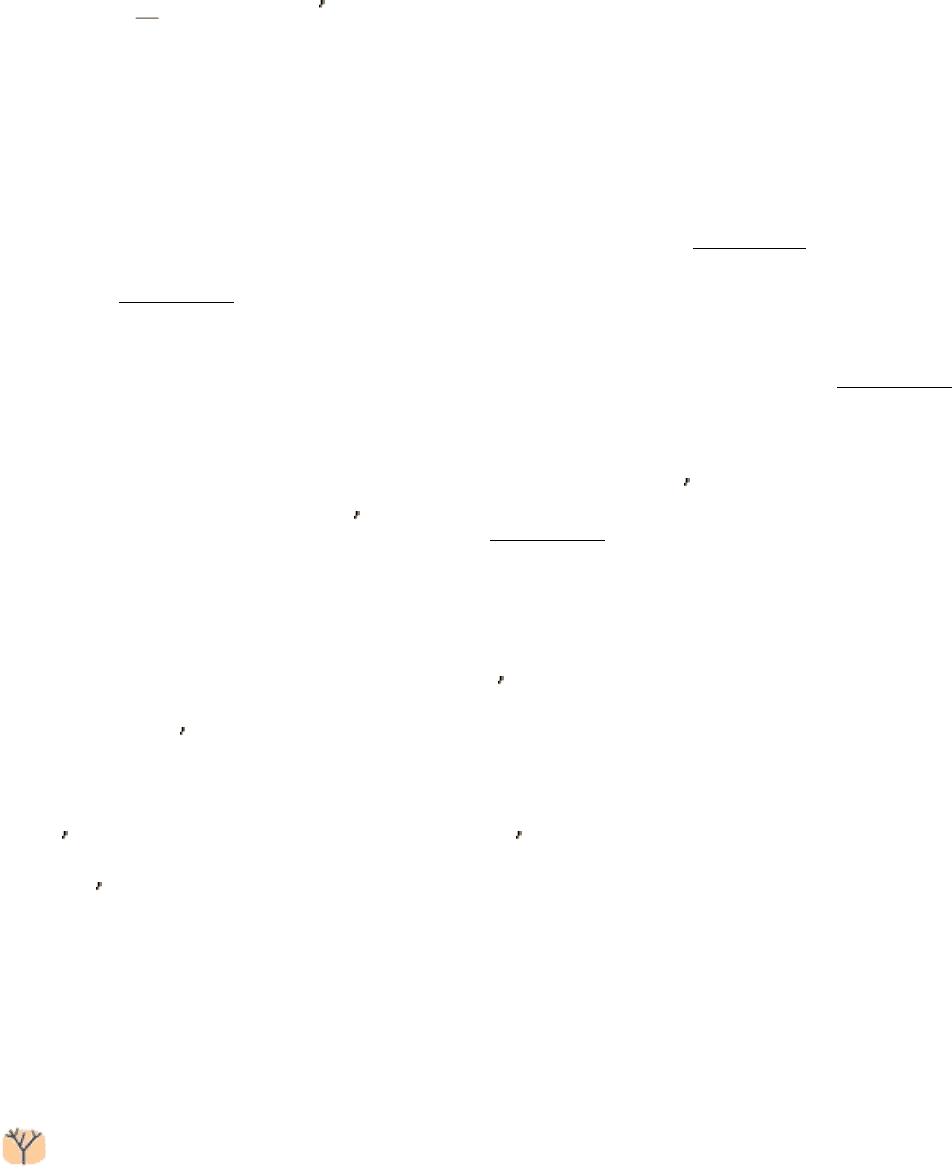
III. Synthesizing the Molecules of Life 25. Nucleotide Biosynthesis
25.3. Deoxyribonucleotides Synthesized by the Reduction of Ribonucleotides
Through a Radical Mechanism
We turn now to the synthesis of deoxyribonucleotides. These precursors of DNA are formed by the reduction of
ribonucleotides
specifically, the 2 -hydroxyl group on the ribose moiety is replaced by a hydrogen atom. The
substrates are ribonucleoside diphosphates or triphosphates, and the ultimate reductant is NADPH. The enzyme
ribonucleotide reductase is responsible for the reduction reaction for all four ribonucleotides. The ribonucleotide
reductases of different organisms are a remarkably diverse set of enzymes. The results of detailed studies have revealed
that they have a common reaction mechanism, and their three-dimensional structural features indicate that these enzymes
are homologous. We will focus on the best understood of these enzymes, that of E. coli living aerobically. This
ribonucleotide reductase consists of two subunits: R1 (an 87-kd dimer) and R2 (a 43-kd dimer).
The R1 subunit contains the active site as well as two allosteric control sites (Section 25.4). This subunit includes three
conserved cysteine residues and a glutamate residue, all four of which participate in the reduction of ribose to
deoxyribose (Figure 25.10).
The R2 subunit's role in catalysis is to generate a remarkable free radical in each of its two chains. Each R2 chain
contains a stable tyrosyl radical with an unpaired electron delocalized onto its aromatic ring (Figure 25.11). This very
unusual free radical is generated by a nearby iron center consisting of two ferric (Fe
3+
) ions bridged by an oxide (O
2-
)
ion.
In the synthesis of a deoxyribonucleotide, the hydroxyl group bonded to C-2
of the ribose ring is replaced by H, with
retention of the configuration at the C-2
carbon atom (Figure 25.12).
1. The reaction begins with the transfer of an electron from a cysteine residue on R1 to the tyrosyl radical on R2. The
loss of an electron generates a highly reactive cysteine thiyl radical within the active site of R1.
2. This radical then abstracts a hydrogen atom from C-3 of the ribose unit, generating a radical at that carbon atom.
3. The radical at C-3 promotes the release of the hydroxide ion on the carbon-2 atom. Protonated by a second cysteine
residue, the departing hydroxide ion leaves as a water molecule.
4. A hydride ion (a proton on two electrons) is then transferred from a third cysteine residue to complete the reduction of
the C-2 position, form a disulfide bond, and reform a C-3 radical.
5. This C-3 radical recaptures the same hydrogen atom originally abstracted by the first cysteine residue, and the
deoxyribonucleotide is free to leave the enzyme.
6. The disulfide bond generated in the enzyme's active site is then reduced by specific disulfide-containing proteins, such
as thioredoxin, to regenerate the active enzyme.
To complete the overall reaction, the oxidized thioredoxin generated by this process is reduced by NADH in a reaction
catalyzed by thioredoxin reductase.
Ribonucleotide reductases that do not contain tyrosyl radicals have been characterized in other organisms. Instead,
these enzymes contain other stable radicals that are generated by other processes. For example, in one class of
reductases, the coenzyme adenosylcobalamin is the radical source. Despite differences in the stable radical employed,
the active sites of these enzymes are similar to that of the E. coli ribonucleotide reductase, and they appear to act by the
same mechanism, based on the exceptional reactivity of cysteine radicals. Thus, these enzymes have a common ancestor
but evolved a range of mechanisms for generating stable radical species that function well under different growth