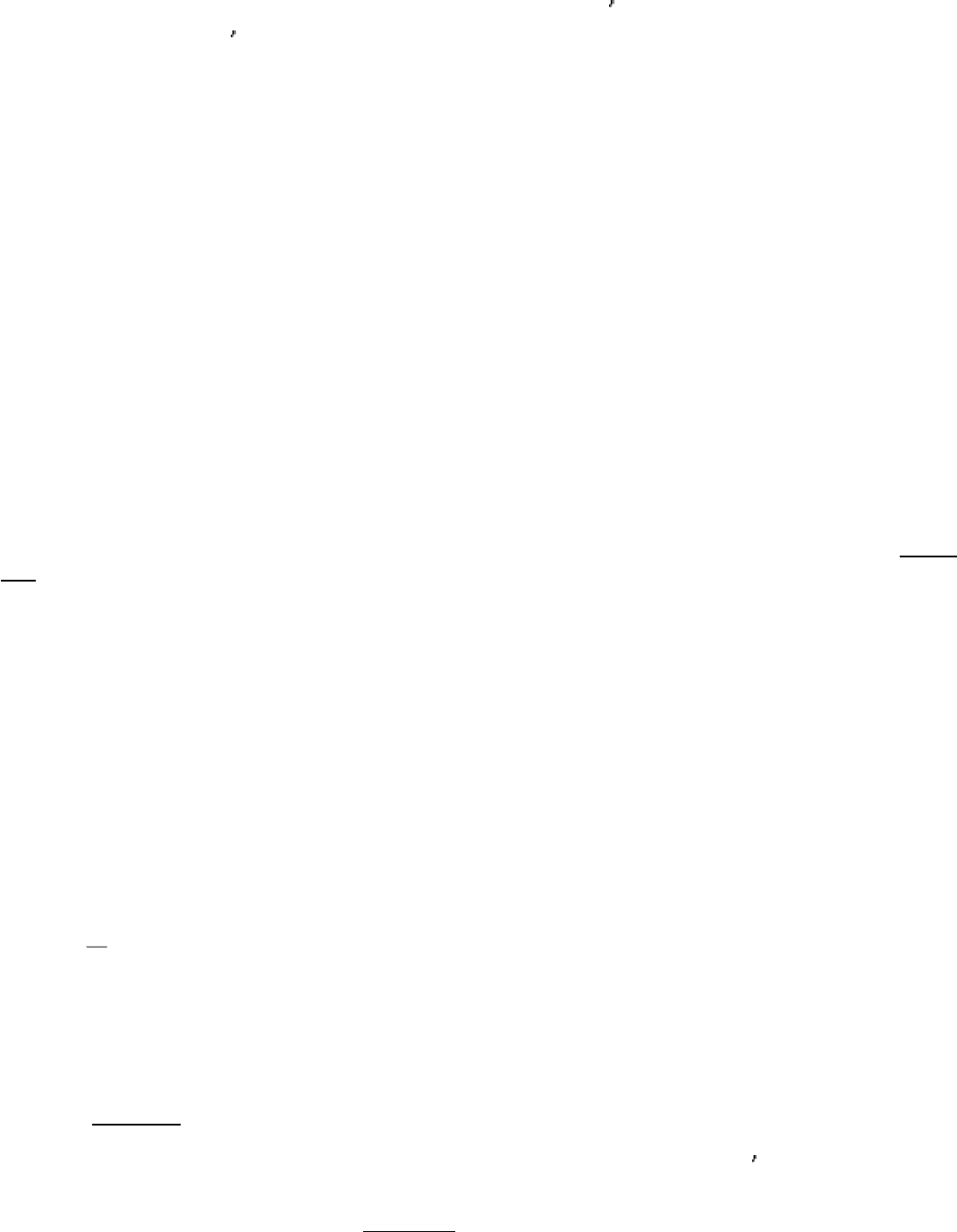
can stimulate transcriptional initiation more than a thousand base pairs away from the start site. Primary transcripts in
eukaryotes are extensively modified, as exemplified by the capping of the 5
end of an mRNA precursor and the addition
of a long poly(A) tail to its 3
end. Most striking is the splicing of mRNA precursors, which is catalyzed by spliceosomes
consisting of small nuclear ribonucleoprotein particles (snRNPs). The small nuclear RNA (snRNA) molecules in these
complexes play a key role in directing the alignment of splice sites and in mediating catalysis. Indeed, some RNA
molecules can splice themselves in the absence of protein. This landmark discovery by Thomas Cech and Sidney Altman
revealed that RNA molecules can serve as catalysts and greatly influenced our view of molecular evolution.
RNA splicing is not merely a curiosity. Approximately 15% of all genetic diseases are caused by mutations that affect
RNA splicing. Morever, the same pre-mRNA can be spliced differently in various cell types, at different stages of
development, or in response to other biological signals. In addition, individual bases in some pre-mRNA molecules are
changed, in a process called RNA editing. One of the biggest surprises of the sequencing of the human genome was that
only approximately 40,000 genes were identified compared with previous estimates of 100,000 or more. The ability of
one gene to encode more than one distinct mRNA and, hence, more than one protein may play a key role in expanding
the repertoire of our genomes.
28.0.1. An Overview of RNA Synthesis
RNA synthesis, or transcription, is the process of transcribing DNA nucleotide sequence information into RNA
sequence information. RNA synthesis is catalyzed by a large enzyme called RNA polymerase. The basic biochemistry of
RNA synthesis is common to prokaryotes and eukaryotes, although its regulation is more complex in eukaryotes. The
close connection between prokaryotic and eukaryotic transcription has been beautifully illustrated by the recently
determined three-dimensional structures of representative RNA polymerases from prokaryotes and eukaryotes (Figure
28.1). Despite substantial differences in size and number of polypeptide subunits, the overall structures of these enzymes
are quite similar, revealing a common evolutionary origin.
RNA synthesis, like nearly all biological polymerization reactions, takes place in three stages: initiation, elongation, and
termination. RNA polymerase performs multiple functions in this process:
1. It searches DNA for initiation sites, also called promoter sites or simply promoters. For instance, E. coli DNA has
about 2000 promoter sites in its 4.8 × 10
6
bp genome. Because these sequences are on the same molecule of DNA as the
genes being transcribed, they are called cis-acting elements.
2. It unwinds a short stretch of double-helical DNA to produce a single-stranded DNA template from which it takes
instructions.
3. It selects the correct ribonucleoside triphosphate and catalyzes the formation of a phosphodiester bond. This process is
repeated many times as the enzyme moves unidirectionally along the DNA template. RNA polymerase is completely
processive a transcript is synthesized from start to end by a single RNA polymerase molecule.
4. It detects termination signals that specify where a transcript ends.
5. It interacts with activator and repressor proteins that modulate the rate of transcription initiation over a wide dynamic
range. These proteins, which play a more prominent role in eukaryotes than in prokaryotes, are called transcrip-tion
factors or trans-acting elements. Gene expression is controlled mainly at the level of transcription, as will be discussed
in detail in Chapter 31.
The fundamental reaction of RNA synthesis is the formation of a phosphodiester bond. The 3
-hydroxyl group of the last
nucleotide in the chain nucleophilically attacks the α-phosphate group of the incoming nucleoside triphosphate with the
concomitant release of a pyrophosphate (see Figure 5.25). This reaction is thermodynamically favorable, and the
subsequent degradation of the pyrophosphate to orthophosphate locks the reaction in the direction of RNA synthesis.