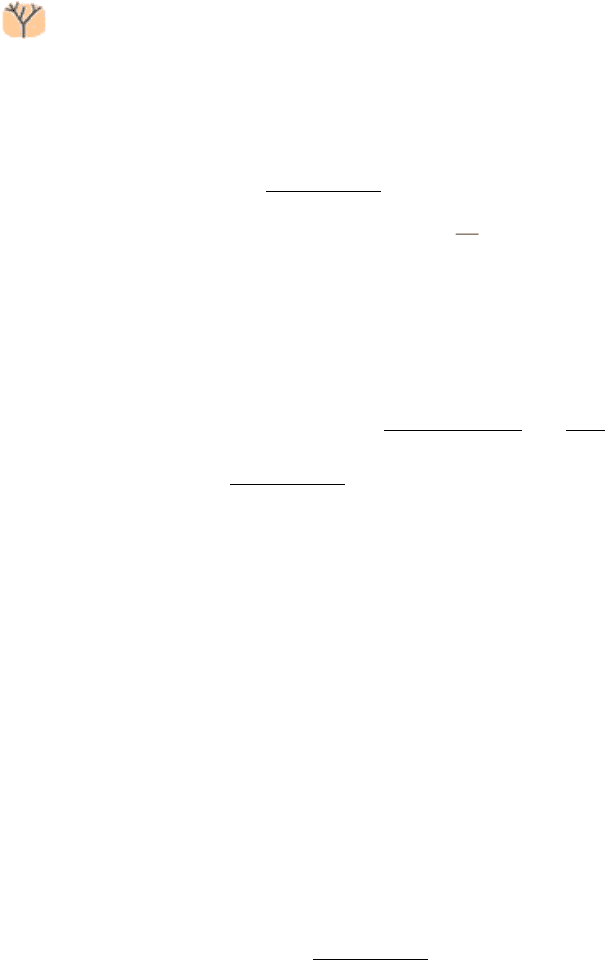
transition from T to R to take place, the bonds between hemoglobin and 2,3-BPG must be broken and 2,3-BPG must be
expelled.
2,3-BPG binding to hemoglobin has other crucial physiological consequences. The globin gene expressed by
fetuses differs from that expressed by human adults; fetal hemoglobin tetramers include two α chains and two γ
chains. The γ chain, a result of another gene duplication, is 72% identical in amino acid sequence with the β chain. One
noteworthy change is the substitution of a serine residue for His 143 in the β chain of the 2,3-BPG-binding site. This
change removes two positive charges from the 2,3-BPG-binding site (one from each chain) and reduces the affinity of
2,3-BPG for fetal hemoglobin, thereby increasing the oxygen-binding affinity of fetal hemoglobin relative to that of
maternal (adult) hemoglobin (Figure 10.24). This difference in oxygen affinity allows oxygen to be effectively
transferred from maternal to fetal red cells. We see again an example of where gene duplication and specialization
produced a ready solution to a biological challenge in this case, the transport of oxygen from mother to fetus.
10.2.4. The Bohr Effect: Hydrogen Ions and Carbon Dioxide Promote the Release of
Oxygen
Rapidly metabolizing tissues, such as contracting muscle, have a high need for oxygen and generate large amounts of
hydrogen ions and carbon dioxide as well (Sections 16.1.9 and 17.1). Both of these species are heterotropic effectors of
hemoglobin that enhance oxygen release. The oxygen affinity of hemoglobin decreases as pH decreases from the value
of 7.4 found in the lungs (Figure 10.25). Thus, as hemoglobin moves into a region of low pH, its tendency to release
oxygen increases. For example, transport from the lungs, with pH 7.4 and an oxygen partial pressure of 100 torr, to
active muscle, with a pH of 7.2 and an oxygen partial pressure of 20 torr, results in a release of oxygen amounting to
77% of total carrying capacity. Recall that only 66% of the oxygen would be released in the absence of any change in
pH. In addition, hemoglobin responds to carbon dioxide with a decrease in oxygen affinity, thus facilitating the release of
oxygen in tissues with a high carbon dioxide concentration. In the presence of carbon dioxide at a partial pressure of 40
torr, the amount of oxygen released approaches 90% of the maximum carrying capacity. Thus, the heterotropic
regulation of hemoglobin by hydrogen ions and carbon dioxide further increases the oxygen-transporting efficiency of
this magnificent allosteric protein.
The regulation of oxygen binding by hydrogen ions and carbon dioxide is called the Bohr effect after Christian Bohr,
who described this phenomenon in 1904. The results of structural and chemical studies have revealed much about the
chemical basis of the Bohr effect. At least two sets of chemical groups are responsible for the effect of protons: the
amino termini and the side chains of histidines β146 and α122, which have pK
a
values near pH 7. Consider histidine
β146. In deoxyhemoglobin, the terminal carboxylate group of β146 forms a salt bridge with a lysine residue in the α
subunit of the other α β dimer. This interaction locks the side chain of histidine β146 in a position where it can
participate in a salt bridge with negatively charged aspartate 94 in the same chain, provided that the imidazole group of
the histidine residue is protonated (Figure 10.26). At high pH, the side chain of histidine β146 is not protonated and the
salt bridge does not form. As the pH drops, however, the side chain of histidine β146 becomes protonated, the salt bridge
with aspartate β94 forms, and the quaternary structure characteristic of deoxyhemoglobin is stabilized, leading to a
greater tendency for oxygen to be released at actively metabolizing tissues. No significant change takes place in
oxyhemoglobin over the same pH range.
Carbon dioxide also stabilizes deoxyhemoglobin by reacting with the terminal amino groups to form carbamate groups,
which are negatively charged, in contrast with the neutral or positive charges on the free amino groups. The amino
termini lie at the interface between the α β dimers, and these negatively charged carbamate groups participate in salt-
bridge interactions, characteristic of the T-state structure, which stabilize deoxyhemoglobin's structure and favor the
release of oxygen.