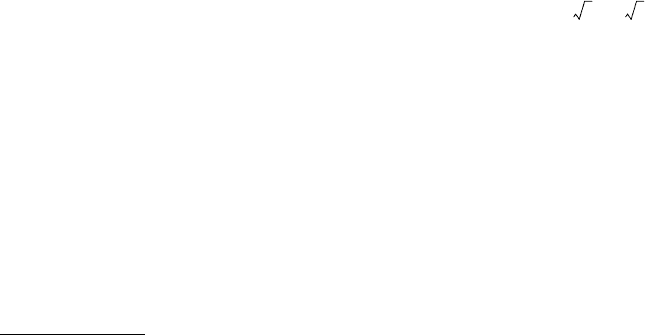
© 1999 by CRC Press LLC
and deformation are minimized. Cobalt, which has a hexagonal close-packed structure, has easy slip
planes and thus transferred in all cases. Thus, simple explanations based on cohesive and interfacial
energies can be misleading if mechanical properties are not taken into account.
We give a second example by Pepper (1974) demonstrating the care necessary in performing studies
of polymer films transferred from polymer pins sliding on a S-Monel disk in ultrahigh vacuum.
Figure 3.26 shows the AES spectra for polytetrafluoroethylene (PTFE), polyvinyl chloride (PVC) and
polychlorotrifluoroethylene (PCTFE) pins sliding on S-Monel. The PTFE spectrum shows large fluorine
and carbon peaks and large attenuation of the metal peaks (care had to be taken due to electron
bombardment desorption of the fluorine). The friction coefficient was low and smooth suggesting slip.
The combined results indicated that PTFE strands were transferring to the metal surface consistent with
the models of Pooley and Tabor (1972). For PVC the AES spectrum shows a large chlorine peak and
small attenuation of the metal peaks suggesting decomposition and chlorine adsorption rather than
polymer transfer. The friction coefficient, although reduced, remained large and exhibited some stick
slip. For PCTFE the spectrum shows chlorine, carbon, and intermediate attenuation of the metal peaks,
but with stability under electron bombardment suggesting the possibility of both decomposition and
some polymer transfer. The friction coefficient was high with stick slip. Consequently, it is difficult to
anticipate what is happening, again demonstrating the need for more extensive surface characterization.
3.6.1 Monolayer Effects in Adhesion and Friction
The bulk of the discussion in this section will be based on the recent high-quality experiments of Gellman
and collaborators (Gellman, 1992; McFadden and Gellman, 1995a,b), while we acknowledge the pio-
neering contributions of Buckley and the members of his group (Buckley, 1981). Gellman has performed
a number of adhesion and friction experiments on single crystals in contact, both for clean and adsorbate
covered interfaces, namely, Cu(111)–Cu(111) and Ni(100)–Ni(100). The apparatus for the friction and
adhesion experiments is shown in Figure 3.27 (Gellman, 1992).
The contacting crystals had a slight curvature in order to prevent contact at the edges of the samples
where large concentrations of steps were expected, and to ensure point contact. The vacuum system was
also vibration isolated which is very important for reasons to be discussed below. Normally, the experi-
ments were repeated for ten different points. The normals of the crystals were aligned by lasers. The
apparatus provided the ability to sputter clean both contacting surfaces, and LEED and AES could be
performed on the surfaces in order to guarantee the crystallinity and to measure contamination and
determine concentration of adsorbates. The AES measurements were performed with LEED optics in
the old retarding field analyzer mode. Following sputter cleaning, the samples were annealed and analyzed
with LEED in order to verify the crystal structure and with AES to verify cleanliness.
On Cu(111) McFadden and Gellman (1995a) examined the effects of sulfur adsorption, since sulfur
is a common antiwear additive. It is often used along with phosphorous in order to prevent metal to
metal contact when the lubricant breaks down. Both surfaces were dosed with sulfur using hydrogen
sulfide, which decomposes upon heating, desorbing the hydrogen and leaving sulfur behind on the
surface. At saturation, sulfur forms an ordered superlattice which has a × R19 degree LEED
pattern. This corresponds to a close-packed monolayer of sulfide ions (S
2–
) on top of the Cu(111) lattice
with an absolute coverage of 0.43 monolayers relative to the Cu(111) substrate. The results of the adhesion
experiments are shown in Figure 3.28.
The adhesion coefficient, µ
ad
= F
ad
/F
N
, which is the ratio of pull-off force to normal force was found
to be 0.69 ± 0.21. As little as 0.05 monolayers (11% of saturation) gave a substantial percentage reduction.
The saturation value at one monolayer was 0.26 ± 0.07. They also found that there was no dependence
of the adhesion coefficient on contact time, separation time, temperature, or normal force. There would
be no expected dependence on normal force since higher loads would simply increase the contact area,
and the adhesion coefficient, therefore, would be expected to track with the normal force. Buckley (1981)
reported adhesion coefficients much greater than 1.0 for clean single crystals in contact. As mentioned
earlier, it is important to control vibrations in the experiments. Bowden and Tabor (1964) showed that
7