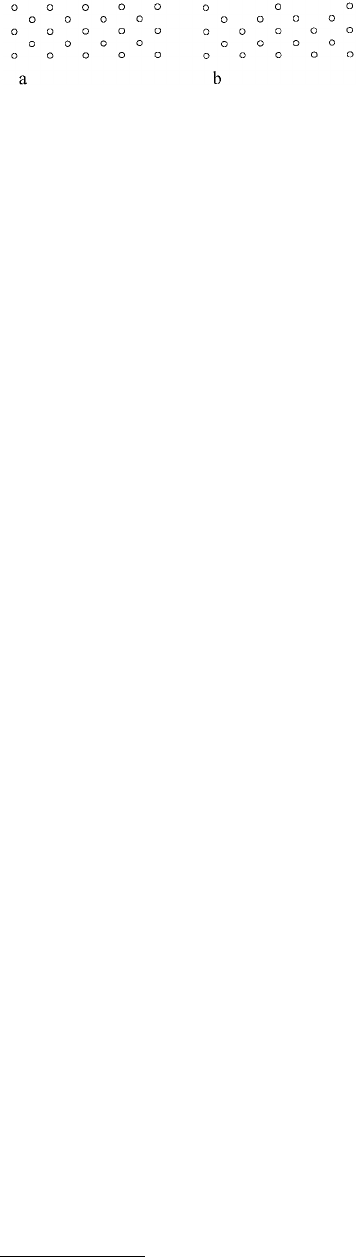
© 1999 by CRC Press LLC
to this behavior where the interplanar spacing increases between the first two layers due to bonding
effects (Needs, 1987; Feibelman, 1992). However, the pattern shown in Figure 3.4 is the usual behavior
for most metallic surfaces. There can be similar changes in position within the planes; however, these
are usually small effects (Rodriguez et al., 1993; Foiles, 1987). In Figure 3.5, we show a side view of a
gold (110) surface (Good and Banerjea, 1992). Figure 3.5a shows the unreconstructed surface and Figure
3.5b shows a side view of the (2
×
1) missing row reconstruction. Such behavior indicates the complexity
that can arise even for metal surfaces and the danger of using ideas which are too simplistic, since more
details of the bonding interactions are needed in this case and those of Needs (1987) and Feibelman
(1992).
Crystal surfaces encountered typically are not perfectly oriented nor atomically flat. Even “on-axis”
(i.e., within a fraction of a degree) single-crystal low-index faces exhibit some density of crystallographic
steps. For a gold (111) face tilted one half degree toward the (011) direction, evenly spaced single atomic
height steps would be only 27 nm apart. Other surface-breaking crystal defects such as screw and edge
dislocations may also be present, in addition to whatever surface scratches, grooves, and other polishing
damage which remain in a typical single-crystal surface. Surface steps and step kinks would be expected
to show greater reactivity than low-index surface planes. During either deposition or erosion of metal
surfaces, one expects incorporation into or loss from the crystal lattice preferentially at step edges. More
generally on simple metal surfaces, lone atoms on a low-index crystal face are expected to be most mobile
(i.e., have the lowest activation energy to move). Atoms at steps would be somewhat more tightly bound,
and atoms making up a low-index face would be least likely to move. High-index crystal faces can often
be thought of as an ordered collection of steps on a low-index face. When surface species and even
interfaces become mobile, consolidation of steps may be observed. Alternating strips of two low-index
crystal faces can then develop from one high-index crystal plane, with lower total surface energy but
with a rougher, faceted topography. Much theoretical and experimental work has been done over the last
decade on nonequilibrium as well as equilibrium surface morphology (e.g., Redfield and Zangwill, 1992;
Vlachos et al., 1993; Conrad and Engel, 1994; Bartelt et al., 1994; Williams, 1994; Kaxiras, 1996).
Semiconductors and insulators generally behave differently. Unlike most metals for which the electron
gas to some degree can be considered to behave like a fluid, semiconductors have strong directional
bonding. Consequently, the loss of neighbors leaves dangling bonds which are satisfied in ultrahigh
vacuum by reconstruction of the surface. The classic example of this is the silicon (111) 7
×
7 structure,
where rebonding and the creation of surface states gives a complex structure. Until STM provided real-
space images of this reconstruction (Binnig et al., 1983) much speculation surrounded this surface.
Zangwill (1988) shows both the terminated bulk structure of Si(111) and the relaxed 7
×
7 structure. It
is clear that viewing a surface as a simple terminated bulk can lead to severely erroneous conclusions.
The relevance to tribology is clear since the nature of chemical reactions between surfaces, lubricants,
and additives can be greatly affected by such radical surface alterations.
There are other surface chemical state phenomena, even in ultrahigh vacuum, just as important as the
structural and bonding states of the clean surface. Surface segregation often occurs to metal surfaces and
interfaces (Faulkner, 1996, and other reviews cited therein). For example, trace quantities of sulfur often
segregate to iron and steel surfaces or to grain boundaries in polycrystalline samples (Jennings et al.,
1988). This can greatly affect results since sulfur, known to be a strong poisoning contaminant in catalysis,
can affect interfacial bond strength. Sulfur is often a component in many lubricants. For alloys similar
geometric surface reconstructions occur (Kobistek et al., 1994). Again, alloy surface composition can vary
dramatically from the bulk, with segregation causing one of the elements to be the only component on
a surface. In Figure 3.6 we show the surface composition for a CuNi alloy as a function of bulk composition
with both a large number of experimental results and some theoretical predictions for the composition
FIGURE 3.5
Side view of gold (110) surface: (a) unrecon-
structed; (b) 1
×
2 missing row surface reconstruction.
(From Good, B. S. and Banerjea, A. (1992),
Mater. Res. Soc.
Symp. Proc.,
278, 211–216. With permission.)