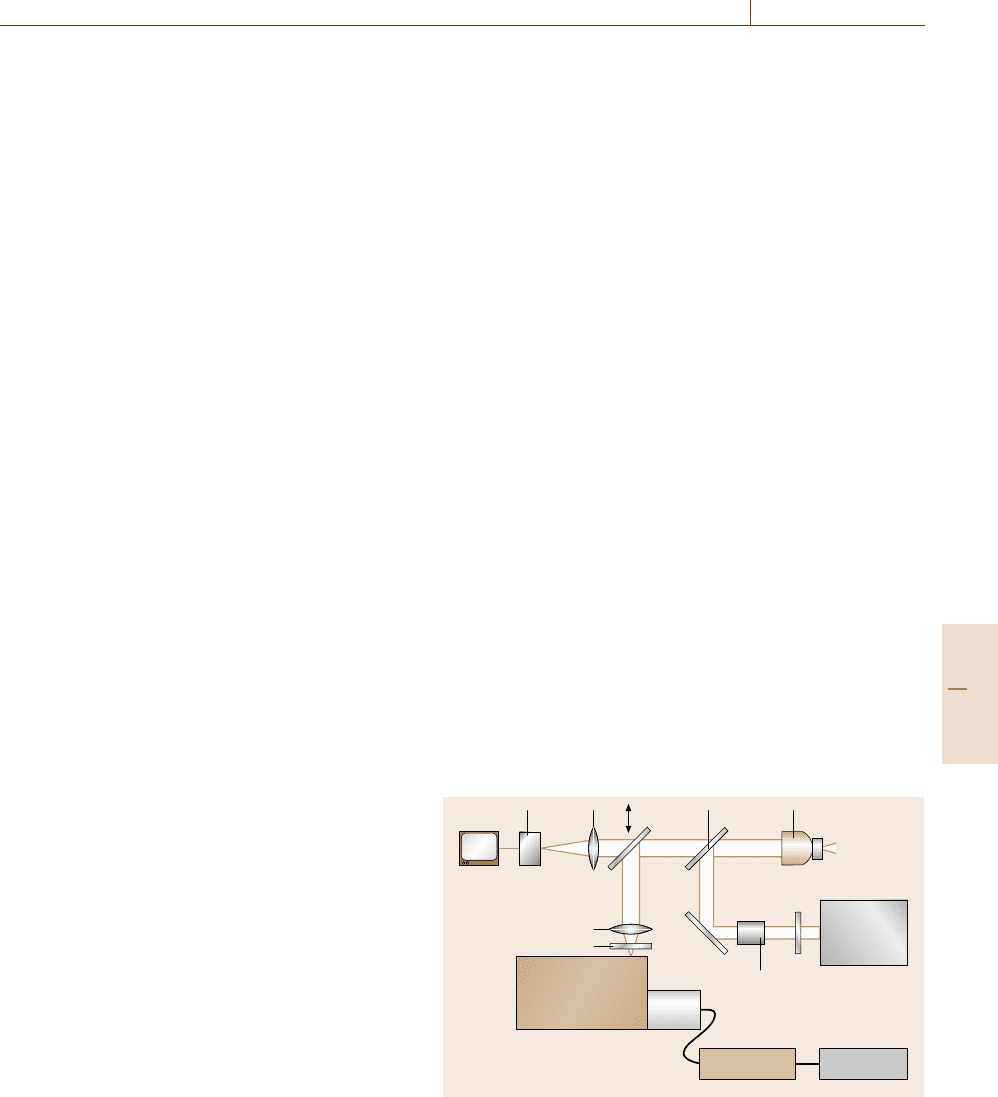
Optical Properties 11.2 Microspectroscopy 605
11.2 Microspectroscopy
In nanoscience and nanotechnology the optical spec-
troscopic study of the individual properties of nanos-
tructured semiconductor materials or biomolecules with
ultrahigh spatial resolution is useful. This is achieved
by avoiding the inhomogeneity caused by differences
in the size, shape or surrounding environment. This
kind of spectroscopy is called single-quantum-dot or
single-molecule spectroscopy. In this section, we will
introduce the principles and the application of three
kinds of microspectroscopic methods based on con-
ventional microscopy, near-field optical microscopy
and cathodoluminescence spectroscopy with the use of
scanning electron microscopy.
11.2.1 Optical Microscopy
Since light has a wave nature and suffers from diffrac-
tion, the spatial resolution of an optical microscope
cannot go below approximately a half of the optical
wavelength: the so-called diffraction limit. The typi-
cal set-up of microphotoluminescence spectroscopy is
illustrated in Fig. 11.21. A laser beam for the photoex-
citation source is focused on a sample surface with
a spot diameter of about 1 μm through an objective
lens with a high magnification factor. The luminescence
from the sample is collected by the same objective lens
and passed through an achromatic beam splitter to sep-
arate the luminescence from the scattered light of the
excitation laser, and the luminescence image is focused
onto a CCD camera or the luminescence spectrum is
analyzed through the combination of spectrometer and
intensified CCD camera.
The principle of single-quantum-dot or single-
molecule spectroscopy is illustrated in Fig. 11.22.For
example, the luminescence from the ensemble of quan-
tum dots of semiconductors having a size distribution
shows the inhomogeneous spectral broadening due to
the size-dependent luminescence peak energy, as shown
in Fig. 11.22a. If the spot size of the focused point is
comparable to the mean separation distance between
the quantum dots, the number of quantum dots de-
tected by the objective lens is limited and the sharp
luminescence lines with discrete photon energies are de-
tected, as shown in Fig. 11.22c. If the distribution of
the dots is dilute enough, one can detect a single dot,
as shown in Fig. 11.22b where the line width is limited
by intrinsic homogeneous broadening corresponding to
the inverse of the phase relaxation time of the excited
state.
As an example of laser microphotoluminescence
spectroscopy, Fig. 11.23 shows the result of the ZnCdSe
quantum dots grown on a ZnSe substrate [11.29]. Al-
though the diameter of the quantum dots is 10 nm on
average and has a wide size distribution, the micropho-
toluminescence spectra show the spiky structures that
critically depend on the spot position of observation.
From the top to the bottom spectra, the spot position
is shifted successively by 10 μm distance. The bottom
spectrum is taken at the original position to check the
reproducibility, from which one notes that the change
in the spectra comes from fluctuation not in time but in
position.
As another example of single-molecule spec-
troscopy, Fig. 11.24 illustrates the microluminescence
excitation spectroscopy for light-harvesting complexes
LH2 acting as an effective light antenna in photosyn-
thetic purple bacteria at 2 K [11.30]. The complexes
contain two types of ring structure of bacterio-
chlorophyll molecules (BChl a) with 9 and 18 mol-
ecules stacked against each other. Since the 9- and
18-molecule rings have their absorption bands at 800
and 860 nm, respectively, the ensemble of LH2 com-
plexes, as illustrated in curve (a), shows two broad
peaks with inhomogeneous broadening caused by dif-
ferent surrounding environment. On the other hand,
when the complexes are dilutely dispersed in polyvinyl
acetate (PVA) polymer film, individual complexes
are found to show different spectra, as illustrated in
curves (b)–(f). Here sharp structures are found around
Sample
Monitor
CCD L2 M2 HM OL
Ti:sapphire
laser
HWPSHG
M1
L1
F
Spectrometer
ICCD
Controller Computer
Fig. 11.21 Experimental set-up of microphotoluminescence spec-
troscopy. HM – dichroic reflection mirror for excitation light; OL
– objective lens; HWP – half-wave plate; F – laser-blocking filter or
linear polarizer
Part C 11.2