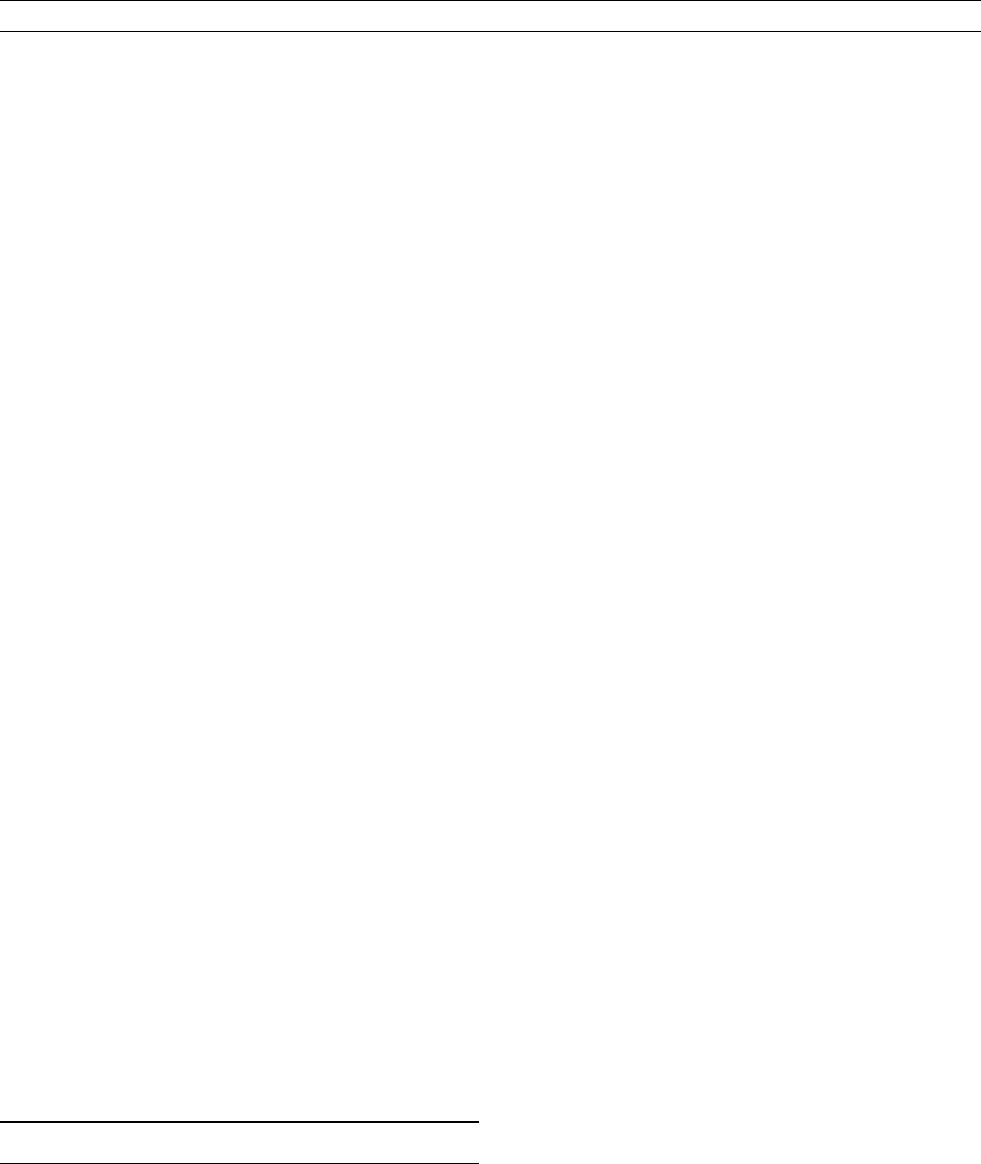
Gamson, P.D., Gibson, I.L., Janecek, T.R., Klootwijk, C.T.,
Lawrence, J.R., Littke, R., Newman, J.S., Nomura, R., Owen,
R.M., Pospichal, J.J., Rea, D.K., Resiwati, P., Saunders, A.D., Smit,
J., Smith, G.M., Tamaki, K., Weis, D., and Wilkinson, C., 1991.
Lower Jaramillo polarity transition records from the equatorial
Atlantic and Indian oceans: Proceedings of the Ocean Drilling Pro-
gram, Scientific Results, 121:377–391.
Hillhouse, J.A., and Cox, A., 1976. Brunhes-Matuyama polarity tran-
sition. Earth and Planetary Science Letters, 29:51–64.
Hoffman, K.A., 1984. A method for the display and analysis of transi-
tional paleomagnetic data. Journal of Geophysical Research, 137:
6285–6292.
Hoffman, K.A., 1991. Long-lived transitional states of the geomag-
netic field and the two dynamo families. Nature, 354: 273–277.
Hoffman, K.A., 1992. Dipolar reversal states of the geomagnetic field
and core-mantle dynamics. Nature, 359: 789–794.
Laj, C., Mazaud, A., Weeks, R., Fuller, M., and Herrero-Bervera, E.,
1991. Geomagnetic reversal paths. Nature, 351: 447.
Love, J.J., and Mazaud, A., 1997. A database for the Matuyama-
Brunhes magnetic reversal. Physics of the Earth and Planetary
Interiors, 103: 207–245.
Mankinen, E.A., Prevot, M., Gromme, C.S., and Coe, R.S., 1985.
The Steens Mountain (Oregon) geomagnetic polarity transition.
I. Directional history, duration of episodes, and rock magnetism.
Journal of Geophysical Research, 90: 10393–10417.
Merrill, R.T., and McFadden, P.L., 1999. Geomagnetic polarity transi-
tions. Reviews of Geophysics, 37: 201–226.
Prevot, M., Mankinen, E.A., Coe, R.S., and Gromme, C.S., 1985. The
Steens Mountain (Oregon) geomagnetic polarity transition. 2. Field
intensity variations and discussion of reversal models. Journal of
Geophysical Research, 90: 10417–10448.
Singer, B.S., and Pringle, M.S., 1996. Age and duration of the
Matuyama-Brunhes geomagnetic polarity reversal for
40
Ar/
39
Ar
incremental heating analyses of lavas. Earth and Planetary Science
Letters, 139:47–61.
Tauxe, L., 1993. Sedimentary records of relative paleointensity of the
geomagnetic field: theory and practice. Reviews of Geophysics, 31:
319–354.
Cross-references
Core-Mantle Boundary Topography, Implications for Dynamics
Core-Mantle Boundary Topography, Seismology
Core-Mantle Boundary, Heat Flow Across Geodynamo
Geodynamo, Dimensional Analysis and Timescales
Geodynamo, Numerical Simulations
Magnetic Anomalies, Marine
Nondipole Field
Reversals, Theory
GEOMAGNETIC POLARITY TIMESCALES
Marine magnetic anomaly record
It is well established that Earth’s magnetic field has alternated fre-
quently but irregularly between two opposing polarity states for at
least most of the Phanerozoic. Older rocks of Proterozoic age that dis-
play both polarity states are also known, but whether they correspond
to reversals of a dipole field is less firmly established. The time inter-
val during which geomagnetic polarity remains constant is called a
polarity chron; long episodes of continuous reversal behavior are
called superchrons. The polarity equivalent to the present state is
referred to as “normal” and the opposite state as “reversed.”
The history of geomagnetic polarity is derived from two sources: the
interpretation of lineated marine magnetic anomalies, and magnetic
polarity stratigraphy in continuous sedimentary sequences and radio-
metrically dated igneous rocks. Since the Late Jurassic, when the cur-
rent phase of seafloor spreading began, these records support and
confirm each other. The geomagnetic polarity timescale (GPTS) is
most reliable for this time. It is divided into two sequences of alternat-
ing polarity, the younger covering the Late Cretaceous and Cenozoic,
and the older corresponding to Early Cretaceous and Late Jurassic
time. The reversal sequences are referred to as the C-sequence and
M-sequence, respectively. They are separated in the oceanic record
by the Cretaceous Quiet Zone in which lineated magnetic anomalies
are absent. It appears that Earth’s magnetic field did not reverse polar-
ity during this time interval, which is referred to as the Cretaceous nor-
mal polarity superchron (CNPS). Prior to the Late Jurassic only the
polarity record preserved in rocks is available. Knowledge of older
geomagnetic polarity history is patchy and, despite some excellent
magnetostratigraphic results, largely unconfirmed.
Construction and identification of a polarity
chron sequence
Although the pioneering studies of geomagnetic polarity were carried
out on radiometrically dated lavas, the marine magnetic anomaly
record provides the most extensive, detailed and continuous record
of reversal history and forms the basis of all GPTS covering the last
160 Ma of the Earth history. The appearance of lineated large ampli-
tude, long wavelength marine magnetic anomalies (Figure G31)
depends on the latitude and direction of the corresponding measure-
ment profile. The first step in constructing a GPTS thus consists of
interpreting the anomalies as a block model of alternating magnetiza-
tion of the oceanic crust responsible for the anomalies. The polarity
pattern is used to correlate coeval segments of different profiles and
to obtain an optimized model that minimizes local variations in spread-
ing rate on any given profile. The ensuing composite block model con-
stitutes a polarity sequence in which the distances between the block
boundaries are proportional to the relative lengths of the polarity
chrons. No consideration is usually given to the finite duration of a
polarity transition, which is thought to last about 4–6 ka (Clement
and Kent, 1984). This time is included in the lengths of polarity
chrons, which are measured between the midpoints of polarity transi-
tions. This simplification is generally acceptable but it may be proble-
matic for accurately describing short polarity chrons for which the
transitional time may be an appreciable fraction of the duration of
the chron.
The anomalies are identified by numbering them in increasing order
away from the spreading axis. In the C-sequence the positive magnetic
anomalies, corresponding to normal polarity, are numbered and pre-
ceded by the letter “C,” as anomalies C18, C29, etc. The M-sequence
oceanic crust in the North Pacific Ocean was magnetized south of the
equator but plate motion has brought it into the northern hemisphere.
The positive anomalies, identified by the letter “M” as anomalies
M0, M13, etc., correspond to reversely magnetized crust. To reconcile
the two numbering schemes, adjacent normally and reversely polarity
chrons are paired, so that the younger member has normal polarity.
Thus marine magnetic anomaly C15 is associated with polarity chrons
C15n and C15r.
To obtain an optimized global model of polarity, which then
becomes the polarity sequence for a GPTS, block models for different
spreading centers must be compared, stretched, and squeezed differen-
tially. The matching of block models may be visual or more sophisticated.
To form an optimized record of the C-sequence of polarity Cande and
Kent (1992a) used nine rotation poles to stack polarity records on contem-
poraneous profiles and determine relative widths of crustal blocks for the
modeled reversal sequence. Before a timescale can be generated, the opti-
mized polarity sequence must be dated. Biostratigraphic stage boundaries
or other dated levels are correlated by magnetostratigraphy to the polarity
328 GEOMAGNETIC POLARITY TIMESCALES