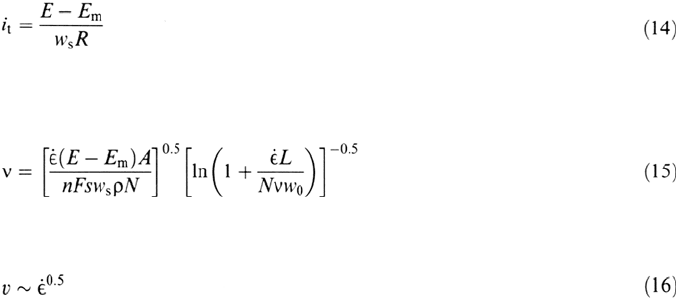
w
s
. Historically, this is an important issue, as these concerns were partly responsible
for the abandonment of the slip-dissolution model by Galvele [20,118].
For a resistive system, the crack-tip anodic current density i
t
can be written
Stress-Corrosion Cracking Mechanisms 425
where E is the potential at the external surface, E
m
is the mixed (corrosion) potential
of the straining crack tip, and R is the electrolyte resistance in the crack. The crack
velocity can be derived on this basis as
As the log term is nearly a constant, this equation explains the commonly observed
relationship [29]
without considering any of the details of the i(t) transient. It would be valuable to
incorporate some of these crack geometry considerations into more rigorous
electrochemical crack models [119].
Type B SCC includes a large number of passive systems in which cracking is
induced by chloride ions. All these systems share an acid crack environment and an
active or semiactive state at the crack tip. For a while it was thought that Al-Li-Cu-
Mg alloys might be an exception to this rule because alkaline crack chemistries are
possible under special conditions (little or no cathode area outside the crack), but
it soon became clear that rapid cracking in this system is associated with acidification
in the crack [120,121].
A fundamental requirement in type B SCC is that the crack velocity must be
able to exceed the localized corrosion velocity; otherwise the crack will degenerate
into a blunt fissure [68] (Figs. 26 and 27). This leads us to expect SCC over a
range of potentials between the repassivation potential of the localized corrosion
process (E
R
) and a higher potential (E
u
) at which the localized corrosion velocity
equals the crack velocity at the prevailing stress intensity value. By manipulating
the composition of a susceptible austenitic stainless steel (Ni, P, Mo, Cu, Al), these
potentials can be made to coincide, guaranteeing immunity to SCC [111,112]. An
increase in temperature is then required to increase E
u
sufficiently for SCC to
occur; i.e., the manipulation of alloy composition increases the critical temperature
for SCC initiation from a crevice. All the applicable alloying elements alter both
the SCC velocity and the crevice corrosion kinetics, so a complete analysis would
be quite complicated.
It is evident that type B SCC rarely occurs by the slip-dissolution mechanism.
The metal in the crack is already in an essentially active state, although there may
be a porous oxyhydroxide layer and possibly a dealloyed metallic layer [78,79]. If
SCC does occur by anodic dissolution in such a system, it is of the trivial kind seen
in sensitized stainless steel or underaged Al-Cu alloys [122] in chloride solutions:
the kinetics of an intergranular pitting process are simply enhanced by the opening
of the crack, reducing the ohmic resistance according to Eq. (13).
All type B systems generate hydrogen within cracks, and in some strong alloys,
such as martensitic stainless steels [91], the cracking is obviously due to hydrogen
Copyright © 2002 Marcel Dekker, Inc.