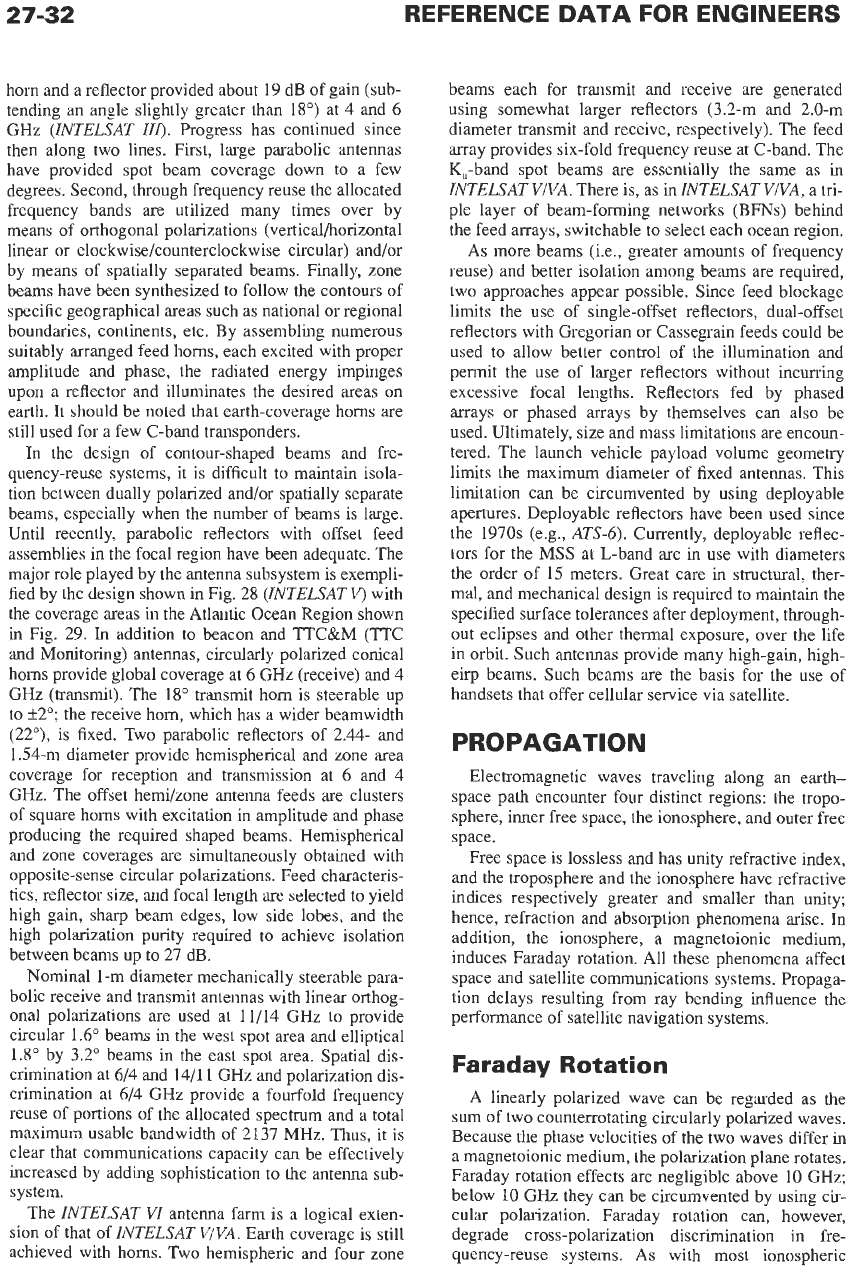
27-32
horn and a reflector provided about 19 dB of gain (sub-
tending an angle slightly greater than
18")
at
4
and
6
GHz
(INTELSAT
HI).
Progress has continued since
then along two lines. First, large parabolic antennas
have provided spot beam coverage down to a few
degrees. Second, through frequency reuse the allocated
frequency bands are utilized many times over by
means of orthogonal polarizations (vertical/horizontal
linear or
clockwise/counterclockwise
circular) and/or
by means of spatially separated beams. Finally, zone
beams have been synthesized
to
follow the contours of
specific geographical areas such as national or regional
boundaries, continents, etc. By assembling numerous
suitably arranged feed horns, each excited with proper
amplitude and phase, the radiated energy impinges
upon a reflector and illuminates the desired areas on
earth. It should be noted that earth-coverage horns are
still used for a few C-band transponders.
In the design of contour-shaped beams and fre-
quency-reuse systems, it is difficult to maintain isola-
tion between dually polarized and/or spatially separate
beams, especially when the number of beams is large.
Until recently, parabolic reflectors with offset feed
assemblies in the focal region have been adequate. The
major role played by the antenna subsystem is exempli-
fied by the design shown in Fig. 28
(INTELSAT
v)
with
the coverage areas in the Atlantic Ocean Region shown
in Fig. 29.
In
addition to beacon and TTC&M (TTC
and Monitoring) antennas, circularly polarized conical
horns provide global coverage at
6
GHz (receive) and
4
GHz (transmit). The
18"
transmit horn
is
steerable up
to
f2"; the receive horn, which has a wider beamwidth
(22"), is fixed. Two parabolic reflectors of 2.44- and
1.54-m diameter provide hemispherical and zone area
coverage for reception and transmission at
6
and
4
GHz. The offset hemi/zone antenna feeds are clusters
of square horns with excitation in amplitude and phase
producing the required shaped beams. Hemispherical
and zone coverages are simultaneously obtained with
opposite-sense circular polarizations. Feed characteris-
tics, reflector size, and focal length are selected to yield
high gain, sharp beam edges, low side lobes, and the
high polarization purity required to achieve isolation
between beams up to 27 dB.
Nominal 1 -m diameter mechanically steerable para-
bolic receive and transmit antennas with linear orthog-
onal polarizations are used at 11/14 GHz
to
provide
circular 1.6" beams in the west spot area and elliptical
1.8" by 3.2" beams in the east spot area. Spatial dis-
crimination at 6/4 and 14/11 GHz and polarization dis-
crimination at 6/4 GHz provide a fourfold frequency
reuse of portions of the allocated spectrum and a total
maximum usable bandwidth of 2137 MHz. Thus, it is
clear that communications capacity can be effectively
increased by adding sophistication to the antenna sub-
system.
The
INTELSAT
VI
antenna farm is a logical exten-
sion of that of
INTELSAT VIVA.
Earth coverage is still
achieved with horns. Two hemispheric and four zone
beams each for transmit and receive are generated
using somewhat larger reflectors (3.2-m and 2.0-m
diameter transmit and receive, respectively). The feed
array provides six-fold frequency reuse at C-band. The
K,-band spot beams are essentially the same as in
INTELSAT VIVA.
There is, as in
INTELSAT VIVA,
a tri-
ple layer of beam-forming networks (BFNs) behind
the feed arrays, switchable to select each ocean region.
As more beams (Le., greater amounts of frequency
reuse) and better isolation among beams are required,
two approaches appear possible. Since feed blockage
limits the use of single-offset reflectors, dual-offset
reflectors with Gregorian or Cassegrain feeds could be
used
to
allow better control of the illumination and
permit the use of larger reflectors without incurring
excessive focal lengths. Reflectors fed by phased
arrays or phased arrays by themselves can also be
used. Ultimately, size and mass limitations are encoun-
tered. The launch vehicle payload volume geometry
limits the maximum diameter of fixed antennas. This
limitation can be circumvented by using deployable
apertures. Deployable reflectors have been used since
the 1970s (e.g.,
ATS-6).
Currently, deployable reflec-
tors for the
MSS
at L-band
are
in
use with diameters
the order of 15 meters. Great care in structural, ther-
mal, and mechanical design is required to maintain the
specified surface tolerances after deployment, through-
out eclipses and other thermal exposure, over the life
in orbit. Such antennas provide many high-gain, high-
eirp beams. Such beams are the basis for the use of
handsets that offer cellular service via satellite.
PROPAGATION
Electromagnetic waves traveling along an earth-
space path encounter four distinct regions: the tropo-
sphere, inner free space, the ionosphere, and outer free
space.
Free space is lossless and has unity refractive index,
and the troposphere and the ionosphere have refractive
indices respectively greater and smaller than unity;
hence, refraction and absorption phenomena arise. In
addition, the ionosphere, a magnetoionic medium,
induces Faraday rotation. All these phenomena affect
space and satellite communications systems. Propaga-
tion delays resulting from ray bending influence the
performance of satellite navigation systems.
Faraday Rotation
A
linearly polarized wave can be regarded as the
sum of two counterrotating circularly polarized waves.
Because the phase velocities of the two waves differ in
a magnetoionic medium, the polarization plane rotates.
Faraday rotation effects
are
negligible above
10
GHz;
below 10 GHz they can be circumvented by using cir-
cular polarization. Faraday rotation can, however,
degrade cross-polarization discrimination in fre-
quency-reuse systems.
As
with most ionospheric