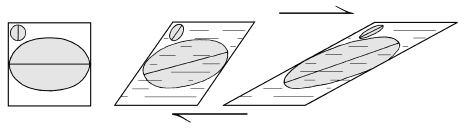
might be posed is how the orientation of the L- and
S-fabric elements—the lineation and foliation in the
tectonite—relate to the principal axes of stress in the
nonhydrostatic stress field that caused the deformation
(Section 8.1). Accurate and complete evaluation of all
three components of deformation is generally im-
possible in real rocks because of the lack of suitable
markers. Furthermore, the observed fabric of a rock
reflects a complex strain history, or deformation path,
that is the sum of all the increments of deformation it
has experienced throughout its entire history, from the
initial to the final state (Box 17.1). If the initial fabric
of the undeformed rock was perfectly homogeneous and
isotropic and the deformation was perfectly coaxial,
then the resulting strain can be homogeneous. But real
rock bodies are almost never isotropic and they are
certainly not homogeneous on most scales; all sorts of
linear and planar discontinuities are mechanically and
rheologically significant in stressed rock. Furthermore,
strictly coaxial deformation is generally deemed to be
uncommon in metamorphic rock systems. Thus, it is a
long interpretive jump from the observed pattern of
the fabric to the state of stress, little of which can be
inferred unambiguously. Principal axes of stress do
not necessarily coincide with the axes of strain ellip-
soids in deformed rocks.
In many instances, the dominant foliation in a meta-
morphic rock is parallel to the plane of flattening in the
ellipsoid (Figures 14.12 and 17.37). Simple flattening
yields only a foliation, as in S-tectonites, and simple
extension (constrictional strain) yields only a lineation,
as in rare L-tectonites. At least two possibilities exist
for widespread L–S tectonites. Foliated and lineated
fabrics that have no evidence for rotational movement,
or have shear-sense indicators in opposite directions,
were possibly produced by pure shear. If, on the other
hand, the L–S tectonite shows a consistent sense of
shear (Figure 17.35), then deformation by simple shear
is likely. In this pattern of ductile flow, the symmetry of
the overall fabric is monoclinic, with the single sym-
metry plane perpendicular to the foliation and to the
axis of rotated crystals and/or parallel to a stretching
lineation, in contrast to the orthorhombic symmetry of
the pure shear fabric.
Only in cases of extreme strain do shear planes
approach parallelism with the plane of flattening in the
strain ellipsoid (Figure 17.37). Foliations are not always
planes of shear, one exception being the C-plane in C–S
fabrics (Figure 17.36).
17.4 ORIGIN OF ANISOTROPIC FABRIC
IN METAMORPHIC TECTONITES
The problematic origin of anisotropic tectonite fabric
has intrigued geologists since the infancy of the science
of geology. The problem is not only petrological but
structural as well because, for example, foliation is
commonly parallel to the axial plane of associated folds
and lineation parallel to hinge lines.
Field and laboratory investigations have shown that
imposed anisotropic fabrics in tectonites result from
penetrative ductile flow in nonhydrostatic stress fields.
Variations in the details of tectonite fabrics imply a
variety of factors affecting their origin, including the
character of the applied nonhydrostatic stress, the
magnitude of the overall strain, the rate of strain, T,
the inherited fabric of the body (whether anisotropic
or isotropic), its grain size, and mineral and chemical
composition, especially the availability of water or
other fluids. Moreover, imposed fabrics can be inho-
mogeneous on different scales, probably reflecting
inhomogeneities in one or more of these factors.
Tectonite fabrics can have any one or more of the
following properties:
1. A preferred dimensional orientation of typically in-
equant mineral grains, such as oriented amphiboles
(Figure 15.8) and oriented mica flakes (Figure
17.29a), as well as flattened and elongate grains of
minerals that are typically equant, such as quartz
and feldspar (Figures 17.14 and 15.17).
2. A preferred orientation of crystallographic axes
and planes, that is, the crystal lattice. In typically
inequant mineral grains, such as amphiboles and
phyllosilicates, a shape orientation as in property 1
automatically, in most cases, ensures a lattice orien-
tation. However, typically equant minerals low in
the crystalloblastic series such as quartz, carbon-
ates, and feldspars can possess strong lattice pre-
ferred orientation without shape orientation, and
vice versa.
3. A compositional layering, expressed usually by
planar variations in concentration of felsic and mafic
minerals (Figure 15.2, see also Figure 19.24a) or of
felsic and phyllosilicate minerals (Figure 15.3).
Current thinking regarding the origin of these three
types of anisotropy is discussed next.
Evolution of Imposed Metamorphic Fabrics: Processes and Kinetics
555
17.37 Progressive deformation by simple shear on surfaces parallel
to the plane of flattening of an initial (large) finite strain ellipse.
The plane of flattening, represented by the maximum axis of
the ellipse, rotates away from the shear surfaces but toward the
plane of flattening of the smaller reference ellipse that shows
the progress of strain in the experiment. Redrawn from Twiss
and Moores (1992).