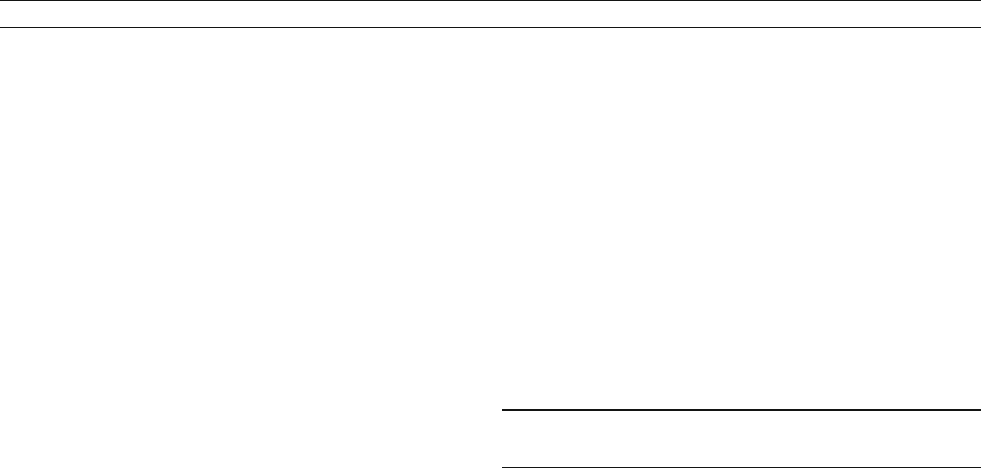
silicate minerals (Reviews in mineralogy, Vol. 31). Washington, D.C.:
Mineralogical Society of America, pp. 463–483.
Ebelmen, J.J., 1845. Sur les produits de la décomposition des espèces
minérales de la famille des silicates. Annales des Mines, 7,3–66.
Edmond, J.M., 1992. Himalayan tectonics, weathering processes, and the
strontium isotope record in marine limestones. Science, 258,
1594–1597.
Elderfield, H., and Schultz, A., 1996. Mid-ocean ridge hydrothermal fluxes
and the chemical composition of the ocean. Annu. Rev. Earth Planet.
Sci., 24, 191–224.
France-Lanord, C., and Derry, L.A., 1997. Organic carbon burial forcing of
the carbon cycle from Himalayan erosion. Nature, 390,65–67.
Galy, A., and France-Lanord, C., 1999. Weathering processes in the
Ganges-Brahmaputra basin and the riverine alkalinity budget. Chem.
Geol., 159,31–60.
Galy, A., France-Lanord, C., and Derry, L.A., 1999. The strontium isotopic
budget of Himalayan rivers in Nepal and Bangladesh. Geochim. Cos-
mochim. Acta, 63, 1905–1925.
Jacobson, A.D., and Blum, J.D., 2003. Relationship between mechanical
erosion and atmospheric CO
2
consumption in the New Zealand South-
ern Alps. Geology, 31, 865–868.
Jenny, H., 1941. Factors of Soil Formation. New York: McGraw-Hill,
281p.
Krishnaswami, S., Trivedi, J.R., Sarin, M.M., Ramesh, R., and Sharma, K.K.,
1992. Strontium isotopes and rubidium in the Ganga-Brahmaputra river
system: Weathering in the Himalaya, fluxes to the Bay of Bengal and
contributions to the evolution of oceanic
87
Sr/
86
Sr. Earth Planet. Sci.
Lett., 109, 243–253.
Lasaga, A.C., Soler, J.M., Ganor, J., Burch, T.E., and Nagy, K.N., 1994.
Chemical weathering rate laws and global geochemical cycles. Geo-
chim. Cosmochim. Acta, 58, 2361–2386.
Milliman, J.D., and Syvitski, P.M., 1992. Geomorphic/ tectonic control of
sediment discharge to the ocean: the importance of small mountainous
rivers. J. Geol., 100, 525–544.
Mora, C.I., Driese, S.G., and Colarusso, L.A., 1996. Middle to Late Paleo-
zoic atmospheric CO
2
levels from soil carbonate and organic matter.
Science, 271, 1105–1107.
Pagani, M., and Freeman, K.H., 1999. Late Miocene atmospheric CO
2
con-
centrations and the expansion of C4 grasses. Science, 285, 876–879.
Palmer, M.R., and Edmond, J.M., 1992. Controls over the strontium
isotope composition of river water. Geochim. Cosmochim. Acta, 56,
2099–2111.
Pearson, P.N., and Palmer, M.R., 2000. Atmospheric carbon dioxide con-
centrations over the past 60 million years. Nature, 406, 695–699.
Petch, S.T., Berner, R.A., and Eglinton, T.I., 2000. A field study of the che-
mical weathering of ancient sedimentary organic matter. Org.
Geochem., 31, 475–487.
Prell, W.L., Murray, D.W., and Clemens, S.C., 1992. Evolution and varia-
bility of the Indian Ocean summer monsoon: Evidence from the Wes-
tern Arabian sea drilling program. In Duncan, R.A., Rea, D.K., Kidd,
R.B., von Rad, U., and Weissel, J.K. (eds.), Synthesis of Results from
Scientific Drilling in the Indian Ocean, Washington D.C.: American
Geophysical Union, pp. 447–469.
Quade, J., Cerling, T.E., and Bowman, J.R., 1989. Development of Asian
monsoon revealed by marked ecological shift during the latest Miocene
in northern Pakistan. Nature, 342, 163–166.
Quade, J., Roe, L., DeCelles, P.G., and Ojha, T.P., 1997. The late Neogene
87
Sr/
86
Sr record of lowland Himalayan rivers. Science, 276,
1828–1831.
Raymo, M.E., and Ruddiman, W.F., 1992. Tectonic forcing of late Ceno-
zoic climate. Nature, 359,117–122.
Richter, F.M., Rowley, D.B., and DePaolo, D.J., 1992. Sr isotope evolution
of seawater: The role of tectonics. Earth Planet. Sci. Lett., 109,11–23.
Rowley, D.B., 2002. Rate of plate creation and destruciton: 180 Ma to pre-
sent. Geol. Soc. Am. Bull., 114, 927–933.
Sarin, M.M., Krishnaswami, S., Dilli, K., Omayajulu, B.l.K., and
Moore, W.S., 1989. Major ion chemistry of the Ganga-Brahmaputra
river system: Weathering processes and fluxes to the Bay of Bengal.
Geochim. Cosmochim. Acta, 53, 997–1009.
Stallard, R.F., and Edmond, J.M., 1983. Geochemistry of the Amazon: 2.
The influence of geology and weathering environment on the dissolved
load. J. Geophys. Res., 88, 9671–9688.
Veizer, J., Ala, D., Azmy, K., Bruckschen, P., Buhl, D., Bruhn, F.,
Carden, G.A.F., Diener, A., Ebneth, S., Godderis, Y., Jasper, T., Korte,
G., Pawellek, F., Podlaha, O.G. and Strauss, H., 1999. Sr-87 /Sr-86,
delta C-13 and delta O-18 evolution of Phanerozoic seawater. Chem.
Geol., 161,59–88.
Walker, J.C.G., Hays, P.B., and Kasting, J.F., 1981. A negative feed back
mechanism for the long-term stabilization of Earth’s surface tempera-
ture. J. Geophys. Res., 86, 9776–9782.
White, A.F., and Blum, A.E., 1995. Effects of climate on chemical weath-
ering in watersheds. Geochim.t Cosmochim. Acta, 59, 1729–1747.
Cross-references
Carbon Cycle
Marine Carbon Geochemistry
Mineral Indicators of Past Climates
Mountain Uplift and Climate Change
Paleosols, Pre-Quaternary
Strontium Isotopes
WISCONSINAN (WEICHSELIAN, WU
¨
RM)
GLACIATION
Introduction
The term “Wisconsinan Glaciation” refers to the last major gla-
cial episode that affected North America, more properly referred
to as the Wisconsinan Stage (Black et al., 1973; Fulton, 1989;
Clark and Lea, 1992)(Figure W1). The term should be restricted
to the deposits and events of the Laurentide Ice Sheet (LIS; see
Laurentide Ice Sheet), which extended from the Canadian Arctic
coast to the northern states in the USA, and across the Dakotas
eastward to New England (Figure W2). In this sense, the term is
not properly used to describe events of the Cordilleran Ice Sheet
(see Cordilleran Ice Sheet), which extended from the Alberta
foothills to the coast of British Columbia. Weichselian and
Würm glaciations refer to the last glacial period in Fennoscandia
and the Alps, respectively, although these terms, in addition
to the “Wisconsinan,” are frequently applied to regions well-
removed from the type areas.
The problem in nomenclature stems from efforts to integrate
the mapping of glacial and other sediments associated with cli-
matic variations into the standard American and International
Stratigraphic Code (Hedberg, 1976). Terms such as Wisconsin
Glaciation or Sangamon Interglaciation are “Geologic-climate
units” whereas Wisconsinan Stage is a time-stratigraphic unit
that requires, by definition, upper and lower isochronous bound-
aries throughout the geographic area to which it is applied (Flint,
1971). By contrast, geologic-climate units are not constrained to
be synchronous, which in reality agrees with the efforts to date
the maximum limit of the LIS during its last major glacial
advance (Dyke and Prest, 1987; Dyke et al., 2002). Such efforts
indicate that the Last Glacial Maximum (LGM; Figure W1)isa
global concept and the timing of the maximum of the late Wis-
consinan Glaciation varies around its margins from around 22 to
possibly as young as 13 cal ka
BP (BP = Before Present with “pre-
sent” equal to
AD 1950). Frequently used terms such as the “late
Wisconsin” generally refer to Marine Isotope Stage (MIS) 2
(Figure W1), but the definitions of middle and early Wisconsin
are more varied in their usage and definitions (Fulton, 1989).
The discussion gets convoluted in terms of definitions and
semantics, leading to a lack of simplicity and uniformity in
usage. For example, as a time-stratigraphic term, the Wisconsi-
nan Stage ends at the Pleistocene/Holocene boundary, which
986 WISCONSINAN (WEICHSELIAN, WU
¨
RM) GLACIATION