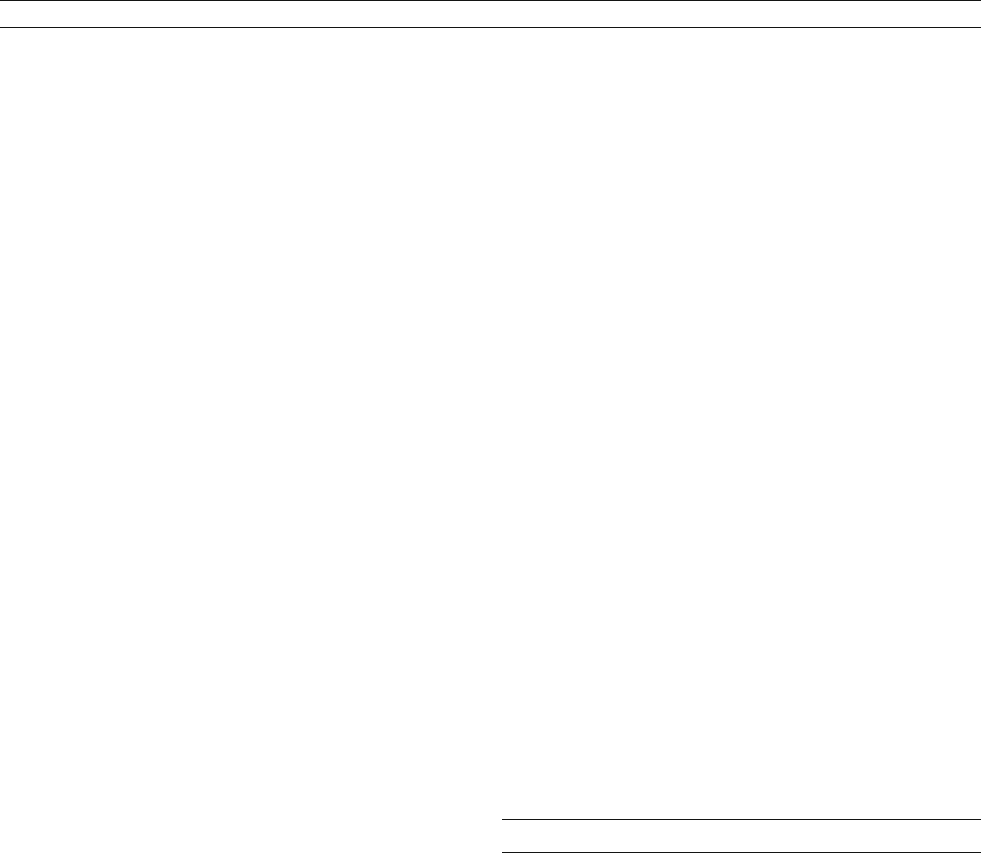
cycle consists of two phases. In the growth phase, the Lauren-
tide Ice Sheet grew through snow accumulation while remain-
ing attached to the bedrock. In the purge phase, the high
pressures caused by the deep ice sheet caused thawing near
the base of the ice sheet thereby allowing the ice sheet to surge
seaward over a lubricated base through Hudson Strait. The
resulting freshwater discharge into the North Atlantic would
be of the order of 0.1–0.2 Sv over a period as short as
250–500 years.
Heinrich events, appearing about every 10,000 years, occur
at the end of a sequence of D-O cycles during a prolonged cold
period. Bond et al. (1993) noted that the sequences of D-O
oscillations tend to follow a saw-tooth cycle (now termed a
Bond Cycle) with successive D-O oscillations involving pro-
gressively cooler interstadials (Figure T5). They argued that
this Bond Cycle was terminated by a Heinrich event, after
which a rapid warming occurred and the process began anew.
The last cold event, known as the Younger Dryas (YD) (also
known as Heinrich event zero, H0) took place between 12,700
and 11,650 years
BP and terminated abruptly within a few
decades. Proxy data suggest that during the YD, even the
AMO was weakened. Further analysis of the proxy record
indicates that the YD was preceded by a warm period in the
North Atlantic climate, known as the Bølling-Allerød (B-A)
warm interval (Figure T5). The B-A interval was accompanied
by a strong global sea-level rise, apparently due to the melting
of continental ice sheets. Until recently, it was not clear how to
reconcile the warm North Atlantic climate during the B-A with
melting of adjacent ice sheets. The latter would have caused a
reduction of AMO, leading to a cooling, rather than warming
of the North Atlantic. A possible explanation lies in the hypoth-
esis that a significant portion of the observed rapid sea level
rise during the B-A could have originated from the melting of
Antarctic ice sheets, rather than solely from melting of the ice
sheets surrounding the North Atlantic. A meltwater pulse into
the Southern Ocean, originating from the partial collapse of
an Antarctic ice sheet, could trigger an enhanced formation of
NADW and an onset of warming in the North Atlantic (Weaver
et al., 2003).
Despite these fundamental advances over the last few years,
many challenges rem ain. In particular, a complete explanation
including the essential role of the MOC, for the existence of
millennial timescale (D-O) variability and its packaging into
Bond Cycles in cold climates, its association with Heinrich
events, and its dependence on the mean climatic state remains
elusive to the pa leoclimate community. Another major climate
mystery is the cause of atmospheric CO
2
and CH
4
changes
during the glacial cycles (Figure T4) and their possible
connection to long -term changes of str ucture and strength of
the GOC.
Andrew J. Weaver and Oleg A. Saenko
Bibliography
Bond, G., Broecker, W., Johnsen, S., McManus, J., Labeyrie, L., Jouzel, J.,
and Bonani, G., 1993. Correlations between climate records from North
Atlantic sediments and Greenland ice. Nature, 365, 143–147.
Dansgaard, W., Johnsen, S.J., Clausen, H.B., Dahl-Jensen, D., Gundestrup,
N.S., Hammer, C.U., Hvidberg, C.S., Steffensen, J.P., Sveinbjörnsdottir,
A.E., Jouzel, J., and Bond, G., 1993. Evidence for general instability of
past climate from a 250-kyr ice-core record. Nature, 364, 218–220.
GRIP Project Members, 1993. Climate instability during the last intergla-
cial period recorded in the GRIP ice core. Nature, 364, 203–207.
Heinrich, H., 1988. Origin and consequences of cyclic ice rafting in the
northeast Atlantic Ocean during the past 130,000 years. Quaternary
Res., 29, 143–152.
Killworth, P.D., 1983. Deep convection in the world ocean. Rev. Geophys.
Space Phys., 21,1–26.
Petit, J.R., Jouzel, J., Raynaud, D., Barkov, N.I., Barnola, J.-M., Basile, I.,
Benders, M., Chappellaz, J., Davis, M., Delayque, G., Delmotte, M.,
Kotlyakov, V.M., Legrand, M., Lipenkov, V.Y., Lorius, C., Pépin, L.,
Ritz, C., Saltzman, E., and Stievenard, M., 1999. Climate and atmo-
spheric history of the past 420,000 years from the Vostok ice core,
Antarctica. Nature, 399, 429–436.
Rintoul, S.R., 1991. South Atlantic interbasin exchange. J. Geophys. Res.,
96, 2675–2692.
Saenko, O.A., Weaver, A.J., and Gregory, J.M., 2003. On the link between
the two modes of the ocean thermohaline circulation and the formation
of global-scale water masses. J. Clim., 16, 2797–2801.
Schmitz, W.J. Jr., and McCartney, M.S., 1993. On the North Atlantic circu-
lation. Rev. Geophys., 31,29–49.
Weaver, A.J., Eby, M., Wiebe, E.C., Bitz, C.M., Duffy, P.B., Ewen, T.L.,
Fanning, A.F., Holland, M.M., MacFadyen, A., Matthews, H.D.,
Meissner, K.J., Saenko, O., Schmittner, A., Wang, H., and Yoshimori,
M., 2001. The UVic Earth System Climate Model: Model description,
climatology and application to past, present and future climates.
Atmosphere-Ocean, 39, 361–428.
Weaver, A.J., Saenko, O.A., Clark, P.U., and Mitrovica, J.X., 2003.
Meltwater pulse 1A from Antarctica as a trigger of the Bølling-Allerød
warm interval. Science, 299, 1709–1713.
Wunsch, C., 2002. What is the thermohaline circulation? Science
, 298,
1179–1181.
Cross-references
Antarctic Bottom Water and Climate Change
Binge-Purge Cycles of Ice Sheet Dynamics
Bølling-Allerød Interstadial
Dansgaard-Oeschger Cycles
Heinrich Events
Millennial Climate Variability
North Atlantic Deep Water and Climate Change
Ocean Paleocirculation
Younger Dryas
TILLS AND TILLITES
Tills, and their lithified equivalents, tillites, are sediments
deposited by glacier ice. In the past, this definition has been
employed in a very broad sense, to include unsorted sediments
deposited in subglacial, supraglacial, ice-marginal, and subaqu-
eous environments. Such a broad definition is difficult to apply
in practice, because it may hard to differentiate sediments
deposited directly from ice and those later reworked by non-
glacial processes. Many researchers now prefer to restrict the
term till to sediments deposited directly by grounded glacier
ice, in subglacial and supraglacial environments, and which
have not undergone significant reworking by fluvial, gravita-
tional, or other processes (Dreimanis, 1989; Benn and Evans,
1998). The non-genetic terms diamict and diamictite have
now generally replaced the older, broad usage of till and tillite.
The correct identification of tills and tillites is important,
because they provide direct evidence for the former distribu-
tion of glaciers, and hence have wide-reaching paleoclimatic
implications.
The main processes of formation of tills are: lodgement,
deformation, melt-out, and sublimation. Lodgement refers to
frictional retardation of clasts or slabs of debris below sliding
948 TILLS AND TILLITES